Authored by Julian L Ambrus*,
Abstract
Purpose: This manuscript reviews the experience of a University Immunology clinic with the evaluation of patients with idiopathic fatigue and exercise intolerance for the presence of metabolic disorders. Laboratory, biochemical and genetic studies were utilized in the evaluation.
Recent Findings: Of the 372 patients evaluated, 95% were found to have a treatable metabolic disorder. A defect in the glycogen storage pathway was found in 78 patients. Mitochondrial disorders were found in 258 patients. Myoadenylate deaminase deficiency was found in 7 patients. Various congenital myopathies were identified in 11 patients. Inflammatory myopathies were identified in 25 patients, 6 of whom had normal muscle enzymes on the initial evaluation.
Summary: The majority of patients (95%) referred with idiopathic fatigue and exercise intolerance after extensive evaluations were found to have underlying metabolic dysfunction. Frequently associated problems included gastrointestinal dysmotility disorders, recurrent infections, Raynaud’s, migraine headaches and various autoimmune diseases. Most patients showed symptomatic improvement with treatment of their metabolic dysfunction.
Keywords: Carnitine palmitoyl transferase; Mitochondria; Glycogen Storage Disease, Myoadenylate Deaminase, Asthma; Sjogren’s syndrome; Raynaud’s; Gastrointestinal dysmotility
Introduction
The last 20 years have seen dramatic increases in medical knowledge that have changed the way that we look at various clinical disorders. One of these advances has been the appreciation that metabolism is a critical part of the functioning of every cell, tissue and organism. Metabolism is defined in the Oxford dictionary as “the chemical processes that occur within a living organism that maintain life”. Metabolism is critical for the production of adenosine triphosphate (ATP) that is necessary to drive many chemical reactions. It is involved with the production of necessary DNA, RNA and proteins. It produces various chemical messengers that are critical for communication among cells and tissues. It determines structural changes that are necessary for cell movement and function. It determines the nature of cellular behavior. Not surprisingly, metabolism is part of everything a person does and therefore alterations in metabolism have clinical implications [1]. Metabolic changes can result from inherited mutations in particular genes regulating metabolism but can also occur because of various environmental and nutritional challenges, including dietary changes, changes in temperature, changes in oxygen concentration and local or systemic inflammation that may occur because of infections or toxic environmental substances [2- 6]. Many medications influence metabolism [7-11].
The first inherited metabolic disorders were identified in young children who often had mutations in genes affecting metabolism that lead to early death [12]. More recently, it has been appreciated that primary or secondary metabolic disorders can present in adults and have a more benign prognosis [13,14]. In adults, the most common presentations for metabolic disorders are fatigue and exercise intolerance [15,16]. Other common symptoms are heat intolerance, difficulty with particular medications, such as statins, metformin and propofol, recurrent infections, bowel dysmotility disorders and accelerated osteoarthritis [17-22].
When evaluating fatigue, one has to consider sleep disorders, endocrine disorders, nutritional disorders, chronic infectious disorders, autoimmune/inflammatory disorders, cardiac disorders, respiratory disorders, psychiatric disorders, malignancies and drug reactions. Recently, adult onset metabolic disorders have been included in this differential diagnosis [23,24]. In fact, fibromyalgia and chronic fatigue syndrome have been shown to be related to metabolic disorders in particular patients [25-29].
This manuscript describes the identification of metabolic disorders in a population of 372 patients referred to a University Immunology clinic over a period of 20 years(1998-2019) by primary care doctors, rheumatologists and other medical specialists after their work up had failed to identify a cause for life altering fatigue and exercise intolerance. Many of these patients had been sent to psychiatrists with the hope that depression or another psychiatric disorder would explain their clinical findings. As we will describe below, 95% of these patients were found to have some form of treatable metabolic dysfunction. Treatment of the identified metabolic disorder lead to clinical improvement in a vast majority of the patients.
Testing
The patients described were all initially evaluated by primary care doctors, rheumatologists and other medical specialists. Profound fatigue and exercise intolerance that had led to life alterations in all patients resulted in the referral to our University clinic. Work up in all patients included complete blood counts, comprehensive metabolic profiles, urinalysis, carnitine, TSH, free T4, CPK, ammonia, lactic acid and in most cases an ischemic forearm test. An elevated lactic acid rest on more than one occasion was interpreted as indicating a reliance on glycolytic metabolism, suggestive of a mitochondrial disorder [30]. Of the patients studied, 169 (46%) had an elevated lactic acid at rest along with 167 (45%) who had an elevated CPK. Serum carnitine was low in only 17 patients (5%). Eighty patients (22%) had a history of thyroid disease, but all had normal thyroid studies at the time of their evaluation in our clinic. Thyroid hormone regulates biogenesis of mitochondria [31]. The ischemic forearm test evaluates changes in lactic acid and ammonia with exercise of the arm causing reliance on anaerobic metabolism. In a normal test, both the lactic acid and ammonia rise 3-4 times the baseline levels. In an adult, a failure of the lactic acid to rise suggests a glycogen storage disease, while a failure of the ammonia to rise suggests myoadenylate deaminase deficiency or a disorder of the urea acid cycle [32,33]. Of the 263 patients who had an ischemic forearm test, 125 were abnormal (48%). In 90 patients, findings were identified in these laboratory studies that allowed a treatment strategy to be initiated and patients did not want any further work up. Eleven patients who did not show clinical improvement with initial treatment and had genetic studies done, which included whole exome sequencing and sequencing of the mitochondrial DNA, done at GeneDx in Gaithersburg, Maryland [34,35].
The remainder of the patients had biochemical studies done from muscle biopsies. The indications for these biochemical studies were normal findings on all the initial laboratory studies, confusing findings on the initial laboratory studies, failure to respond to therapy based on the initial laboratory studies and/or curiosity of the patients to want to identify the root cause for their disorder.
Biochemical studies included the evaluation of the activity of carnitine palmitoyl transferase, myoadenylate deaminase, phosphorylase, phosphorylase b kinase, phosphofructokinase, phosphoglycerate kinase, phosphoglycerate mutase, lactate dehydrogenase, acid and neural maltase, NADH dehydrogenase, NADH cytochrome c reductase, succinate dehydrogenase, succinate cytochrome c reductase, cytochrome c oxidase and citrate synthase from freshly obtained muscle biopsies. In some patients CoQ10 levels were also assessed. These studies were performed in the Robert Guthrie Genetics Laboratory as previously described [8]. Treatment strategies were determined by the biochemical studies, when possible. Some patients with normal biochemical studies in whom no treatment strategy could be suggested went on to have genetic studies.
The histology of the muscle obtained for biochemical studies was evaluated. The vast majority of the biopsies showed only fiber atrophy (67%). Other less common findings included normal (11%), inflammatory myositis (11%), consistent with denervation (2%), Ragged red fibers or abnormal mitochondria on EM (4%), inclusion body myositis (3 patients), abnormal lipid deposition (1 patient), abnormal glycogen deposition (1 patient) and vasculitis (2 patients).
The biochemical studies revealed the following: Mitochondrial Respiratory Chain:
Complex 1 activity <70% - 71/216 (33%)
Complex 1 activity <50% - 10/216 (5%)
Complex 2 activity <70% - 15/216 (7%)
Complex 2 activity <50% - 1/216 (0.5%)
Complex 3 activity <70% - 48/216 (22%)
Complex 3 activity <50% - 13/216 (6%)
Complex 4 activity <70% - 16/216 (7%)
Complex 4 activity <50% - 5/216 (2%)
CoQ10 activity < 100% - 16/72 (22%)
In addition, carnitine palmitoyl transferase activity <65% in 70/216 patients (32%), LDH activity was <20% in 58/216 patients (27%), phosphoglycerate mutase activity was < 50% in 6/216 patients (3%) and myoadenylate deaminase activity was <10% in 7/216 patients (3%).
Ultimately 83 patients had genetic studies. The notable findings were as follows:14 patients had glycogen storage diseases (4-alpha glucosidase deficiency [Pompe disease=glycogen storage disease type II], 3-myophosphorylase deficiency [McArdle’s disease = glycogen storage disease type V], 5-phosphorylase kinase B deficiency [glycogen storage disease type IX], 1 phosphofructokinase deficiency [Tarui disease = glycogen storage disease type VII], 1-beta enolase deficiency [glycogen storage disease type XIII])
25 patients had mutations in various mitochondrial respiratory chain enzymes. 8 patients had mitochondrial depletion mutations (6-DNA polymerase gamma [POLG]; 1-mitochondrial genome maintenance exonuclease 1 [MGME 1]); 1-p53 inducible ribonucleotide reductase [RRM2B]. 12 patients had mutations in other mitochondrial genes (1-uncoupling protein 3 [UCP3], 3-optic atrophy 1 [OPA 1], 2 -very long chain acyl-CoA dehydrogenase [VLCAD], 2-mitofusin 2 [MFN 2], 4-various mitochondrial tRNA). 11 had mutations in various genes associated with congenital myopathies (1-lamin A/C [LMNA], 1- Rapsyn [RAPSN], 1-calsequestrin [CASQ1], 1-Titin [TTN], 2-Kelch repeat and BTB Domain Containing 13 [KBT BD 13; Nemaline myopathy 6], 1-Collagen type VI alpha 2 chain [COL6A2], 3-Ryanodine receptor 1 [RYR 1], 1-regulator factor X, 3 [RFX 3])
5 had mutations in myoadenylate deaminase. 8 had no relevant metabolic gene mutations identified, but 6 had autoinflammatory syndromes ( 1-Tumor necrosis factor receptor -associated periodic syndrome [TRAPS], 1-Nacht, LRR and PYD domains-containing protein 12 [NLRP12] associated cryopyrin associated syndrome, 2-Familial Mediterranean fever, 2-Melvalonate kinase deficiency).
The actual work up done on each individual patient depended upon the findings, how far the patient was interested in pursuing their work up and what their insurance company was willing to pay for. Of the patients who had normal blood studies and went on to have the biochemical studies done, 36 had significant findings on the biochemical studies. There were 29 patients who had abnormal blood studies and went on to have biochemical studies that were normal. Thirteen of these patients had genetic studies that found mutations consistent with their phenotype. There were 11 patients who had normal blood studies, did not want to have a muscle biopsy and had significant findings on their genetic work up. There were 6 patients who had abnormal blood studies but got no further elucidation of their disorder from a combination of biochemical and genetic studies. Overall, most patients arrived at a treatable diagnosis with the combination of the above studies. Figure 1 demonstrates the studies that ultimately led to a treatable diagnosis in this patient population-20% from laboratory studies, 21% from genetic studies and 58% from biochemical studies. Figure 2 demonstrates the diagnoses that were reached in these patients that allowed development of a treatment strategy-78 had abnormalities of an enzyme in the glycogen storage pathway, 168 had abnormalities of a mitochondrial respiratory chain function, 8 had mitochondrial depletion syndromes, 12 had defects in other mitochondrial proteins, 11 had mutations in proteins associated with congenital myopathies, 7 had abnormal myoadenylate deaminase and 70 had low carnitine palmitoyl transferase activity. Of the 372 patients in this manuscript 16 had blood and biochemical studies that did not reveal a treatable diagnosis (and never had genetic studies done) and 2 had blood, biochemical and genetic studies that did not reveal a treatable diagnosis. Therefore only 18 of 372 patients (5%) failed to get a diagnosis by the end of the studies. The majority of these patients showed improvement with treatment.
Treatment
The treatment of mitochondrial dysfunction or mitochondrial respiratory chain disorders involved a combination of medications shown to enhance respiratory chain function and ATP production. CoQ10, which transports electrons between the complex I and complex III of the respiratory chain, folic acid, which is a cofactor for several respiratory chain enzymes, carnitine, which helps import fatty acids into the mitochondria, alpha lipoic acid, which is a strong anti-oxidant and creatine, which generates ATP through the creatine phosphate shuttle [26,36-42]. Patients with low carnitine palmitoyl transferase activity were also put on diets minimizing long chain fatty acids [43,44].
Patients with glycogen storage diseases were placed on diets restricting complex carbohydrates with larger amounts of simple sugars [45,46]. In patients with low activity of lactate dehydrogenase, patients were also given the medications to treat mitochondrial dysfunction, because of the influence of lactic acid on mitochondrial functions [47,48].
Patients with myoadenylate deaminase deficiency were treated with ribose [49]. In addition, they were given the medication for mitochondrial dysfunction because loss of ammonia could lead to depletion of fumarate which is necessary for the normal functioning of the citric acid cycle [32,50], although this affect has been questioned in one study [51].
Doses of these medications were adjusted as needed for individual patients. At the same time, it was necessary to optimize management of other clinical issues that contributed to the patients’ symptoms, such as sleep apnea, food hypersensitivities and Raynaud’s.
Additional Clinical Findings
All of the patients were referred with fatigue and exercise intolerance. Eighty-one percent of the patients carried a diagnosis of fibromyalgia given them by an Internist or Rheumatologist. Eleven percent of the patients were labeled as having difficult to treat depression. Eighteen of the patients (5%) had significant dyspnea, the cause for which could not be identified and was felt to be secondary to respiratory muscle dysfunction. Seventeen percent had been diagnosed with asthma, which is roughly double the incidence in the adult population [52]. Two patients had recurrent bouts of rhabdomyolysis. Twenty-five patients (7%) were ultimately found to have some form of inflammatory myositis. Six of these patients had normal CPK at their initial evaluation and would not have normally been evaluated for an inflammatory process. Interestingly, accelerated osteoarthritis involving the spine, hips and knees was found in 15% of the patients, presumably due to poor muscle function supporting the joints [22,53]. Congestive heart failure was present in 7 patients (2%), possibly related to the effect of a metabolic disorder on heart muscle function.
Interestingly, a majority of the patients (73%) had some form of gastric dysmotility, which included gastroesophageal reflux, gastroparesis, nausea, vomiting, constipation, diarrhea and bouts of pseudo-obstruction. Many patients (45%) had various food hypersensitivities including celiac sprue, so that elimination diets were a critical part of their management. Only 13 patients (3%) had a definitive diagnosis of inflammatory bowel disease. Another very common problem in these patients was recurrent infections (44%). Many of them had been worked up for B and T cell disorders. Eight patients (2%) had hypogammaglobulinemia, which in two cases was related to protein losing enteropathy from food hypersensitivities.
Other very common manifestations were Raynaud’s (37%), migraine headaches (54%) and anti-phospholipid antibodies (15%). Many of the patients with migraine headaches responded to centrally acting vasodilators, so they probably had reversible central nervous system vasospasm, or Raynaud’s of the brain [54-56]. Surprisingly, many of the clinical features described with particular metabolic disorders were not common in this cohort: diabetes (8%), hearing loss (5%), visual loss (3%) and peripheral neuropathy (6%). Many patients did have heat intolerance and had had reactions to propofol, statins or metformin, drugs known to be metabolic poisons [8,10,11].
Other notable clinical findings in these patients included various autoimmune diseases: Sjogren’s syndrome (14%), inflammatory arthritis (10%), scleroderma (2%) and SLE (2%). All of these disorders are present at a much lower frequency in the general population [57-61].
Figure 3 demonstrates the overall clinical manifestations that were most common in this cohort of patients. It provides an important listing of problems that should alert a physician that a metabolic work up is indicated. Furthermore, it provides an important list of problems that go along with metabolic disorders, each of which has to be properly addressed if patients are going to improve symptomatically with their therapy.
Discussion
This manuscript describes a cohort of patients that was referred to a University Immunology clinic because of idiopathic life altering fatigue and exercise intolerance. Additional clinical symptoms, such as gastrointestinal dysmotility, recurrent infections and accelerated osteoarthritis, suggested that there was an underlying systemic problem responsible for the clinical picture. Using a combination of laboratory studies, biochemical studies from muscle biopsies and genetic studies 95% of patients were identified to have a form of metabolic dysfunction that could be improved with available therapies. Treatment was life altering for many of these patients, although improvements in treatments for these disorders is clearly needed.
The recognition of metabolic disorders in adults is relatively recent [45,62-65]. A burgeoning literature identifies metabolic dysfunction as part of the normal aging process [66-68]. At the same time, however, many patients with inherited metabolic disorders found ways to compensate for them so that they are not noticeably symptomatic unless several factors conspired together. For example, patients with inherited metabolic disorders avoided activities that require a lot of energy expenditure, such as competitive sports during school. Patients with glycogen storage diseases had already figured out that they need to avoid complex carbohydrates. Patients with mitochondrial disorders avoided hot climates. Many patients had learned to take naps during the day. When these patients develop infections or other stressors, however, their difficulty in dealing with them made it obvious that something additional was going on.
Even more recent is the appreciation that many metabolic disorders in adults are acquired and may complicate the management of other disease processes. For example, patients with hypoxia related to lung disease or congestive heart failure will develop secondary mitochondrial dysfunction because of the requirement for oxygen in oxidative phosphorylation [69- 70]. Patients with sepsis develop multiple secondary metabolic disorders that can affect cardiac, muscle and immunological function [4,71-73]. Patients with chronic inflammation will damage local mitochondria because of local cytokine and toxin production [74].
At the same time, an underlying metabolic disorder predisposes an individual to have infections because of altered immune function [75,76]. Recurrent infections can cause tissue injury that contributes to bowel dysmotility, food hypersensitivity, autoimmunity and other forms of organ dysfunction [77-79]. Infections can alter the intestinal microbiome leading to further metabolic and immune regulatory problems [80]. Underlying metabolic disorders can alter vascular function, by reducing production of mediators such as nitric oxide, leading to organ dysfunction from altered perfusion [81-84]. Mitochondrial dysfunction has been implicated in the vasculopathy associated with diabetes [81,85,86]. Mitochondrial dysfunction has been observed in migraine headache, whether or not it is primarily vascular in nature [87].
What is emerging from these and similar studies is that metabolic dysfunction is part of many disease processes and needs to be addressed as one of the factors contributing to the pathophysiology of the disorder. For example, studies have demonstrated mitochondrial dysfunction in patients with SLE and improvement in the clinical status of the patients with antioxidants [88,89]. Particular patients with neurodegenerative diseases, such as Parkinson’s disease and multiple sclerosis, have been shown to benefit from treatment of mitochondrial dysfunction [90- 92]. Particular patients with depression see resolution of their symptoms with treatment of an underlying metabolic disorder, if it is present [93,94]. This was observed in many of the patients described in these studies.
Overall, the manuscript seeks to
1) make people aware of the existence of primary and secondary metabolic disorders in adults.
2) identify clinical symptoms and factors that make it more likely that a metabolic disorder will be identified in particular patients.
3) identify strategies for the diagnosis of these disorders. As research progresses to improve treatment of these disorders, the ability to recognize their existence will become more and more important.
Figure Legends
Figure 1
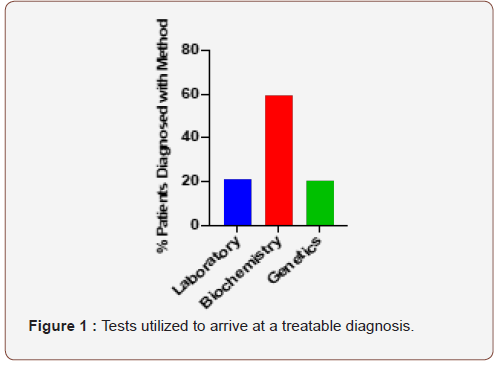
This figure demonstrates the percentage of patients who reached a treatable metabolic diagnosis based on either laboratory studies (20%), biochemical studies (59%) or genetic studies (21%) (Figure 1).
Figure 2
This figure demonstrates the number of patients who were diagnosed with the following disorders: defective glycogen storage pathway [78], defective mitochondrial respiratory chain [68], low carnitine palmitoyl transferase activit[70], mitochondrial depletion syndromes [8], mutations in other mitochondrial genes [12], congenital myopathies [11], myoadenylate deaminase deficiency [7] or no abnormality [18] (Figure 2).
Figure 3
This figure demonstrates percentage of patients expressing the common clinical manifestations of the patients evaluated in this cohort: Fatigue (100%), Exercise Intolerance (100%), Fibromyalgia (81%), Gastrointestinal Motility (73%), Migraine Headaches (54%), Associated Autoimmune Disease (48%; includes Sjogren’s syndrome-14%, Scleroderma-2%, SLE-2 %, Inflammatory arthritis-10%, Inflammatory bowel disease-3% and Antiphospholipid antibody syndrome-15%), Food hypersensitivities (45%), Recurrent infections (44%) and Raynaud’s (37%) (Figure 3).

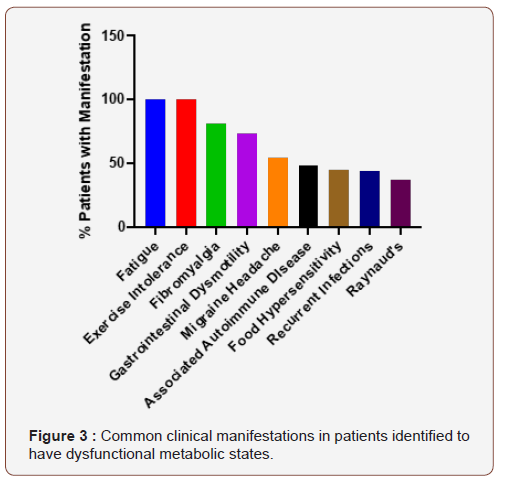
To read more about this article...Open access Journal of Rheumatology & Arthritis Research
Please follow the URL to access more information about this article
To know more about our Journals...Iris Publishers
No comments:
Post a Comment