Authored by Ayodele A Otaiku*,
Abstract
24.95 square kilometers Nigerian military shooting range established in 1965 was studied (June-August 2015 and February-March 2016) for in situ bacteria consortia impacts on xenobiotic biodegradation using polymerase chain reaction amplification of 16srRNA gene. The optical density reading of biodegrading activity of each bacterial isolate on explosives broth with 1% exposure mineral salt medium (MSM) was evaluated. Mixed bacterial consortia (MBC) observed high bioremediation potential (68.62%) compared to single isolate in decreasing order were Bacillus subtilis, 55.1%; Enterobacter spp, 54.80%; Escherichia coli, 54.1%; Arcobacter spp, 43% Klebsiella pneumonia 42.7%, Lysini bacillus 37.8% and Achromobacter spp, 31.5% respectively attributed to the synergistic effect between the catabolic enzymes in the eight bacteria isolates. Anova test for total explosive contents shows a significant difference for all locations in both dry and wet Seasons (P<0.05) according to the Duncan multiple range test. All bacteria isolate reduced heavy metals better than the control in decreasing order were Bacillus subtilis>Enterobacter spp>Escherichia coli>Arcobacter spp>Klebsiella pneumonia >Lysinibacillus >Achromobacter spp. MBC observed high bioremediation potential (68.62%) compared to single isolate. MBC has the highest and prominent percentages reduction on heavy metal pollutants as a consequence of microbial ecology, bacterial strains having plasmid linked degradation or chromosomal genes, reduction ability, substrate specificity influenced microbial remediation of the explosives xenobiotic. Habitat filtering principle and resource ratio theory explains microbial completion and genetic information of more than one organism is necessary to degrade the complex mixtures pollutants as expressed in the MBC biodegradability (Figure 1).
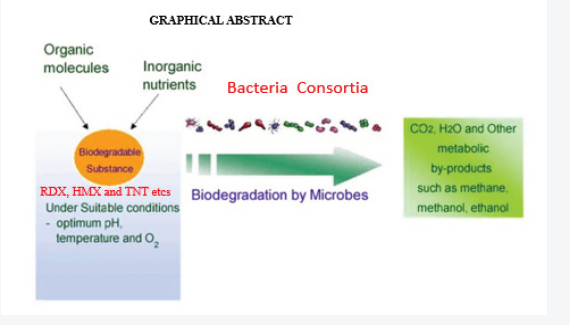
Keywords: Shooting range; 16srRNA; Polymerase chain reaction; Bioremediation; Xenobiotic; Bacterial consortia; Microbial competition; Explosives; Enzymes; Heavy metals; Biodegradation.
Introduction
The manufacture use and disposal of explosives as well as the demilitarization of military facilities can result in environmental contamination. The three most widely used explosives are 2,4,6-trinitrotoluene (TNT), hexahydro-1,3,5-trinitro-1,3,5-triazine
(RDX) and octahydro-1,3,5,7-tetranitro-1,3,5,7-tetrazocine (HMX). Technological advancements in microbial bioremediation include the conversion of slow biodegradation process into a rapid by means of several modern methods including microbial fuel cells (MFCs) [1,2], bioreactors [3], biofilms [4] and the use of microbial consortia for the degradation of recalcitrant organic compounds [5]. In ex-situ bioremediation technologies for explosives such as composting [6-8]. Biological based remediation is an economical and ecological compatible approach to detoxify areas contaminated with xenobiotics [4,9,10]. The reductive reactions are the basis of several treatment processes for the bioremediation of TNT contaminated soils [11-14]. However, TNT is resistant to oxidative microbial degradation and only low mineralization rates have been sporadically reported with bacterial consortia. Instead of oxidation, many bacteria catalyse the reduction of one or two nitro-groups of TNT to mono amino di nitrotoluenes (ADNT) and diamino nitrotoluenes (DANT). Another pathway is mediated by addition of one or two hydride ions to the aromatic ring, resulting in the formation of Meisenheimer-complexes (adducts of aromatic nitro-compounds with a nucleophile) often accompanied by release of nitrite. The electron transfer is catalyzed by different types of cytoplasmatic nitroreductases [15,16]. Also, precursors and metabolites of TNT are classified as very toxic, carcinogenic and mutagenic [17-19]. Typical contaminated sites may contain up to 10 g/kg TNT in soils and up to 100 mg/l in water. TNT and its metabolites exhibit a high toxic and mutagenic potential on both prokaryotes and eukaryotes [20-23]. TNT and some of its degradation products have a high persistence, toxicity and mutagenicity [20]. The objective of the research was to isolates the bacteria organisms in the military shooting range to ascertain their biodegrading capabilities on explosives (RDX, TNT, HMX) and heavy metals contaminants and the impacts of the mixed bacterial consortia (MBC) biodegradability.
Microbial bioremediation
In general, single microbial species hardly degrades any organic substrate in isolation [24] and works well in community. Interactions between microbes in the community ensure the exchange of genetic information between microbial species conferring resistance, tolerance and chemical-degrading ability. These processes are further subjected to the varying environmental conditions influencing the growth pattern of microbes [25] Microbiological removal of metal ions from the environment is a new biotechnique [26] and the most cost-effective approach in mitigation of elemental pollution. It is imperative to know that microbes in any case cannot degrade the metal ions and are only able to transform metallic ions from higher to lower oxidation states to stabilize them [27]. Successful microbial bioremediation is achieved when microbes interact within their niche, under the most favourable conditions [28]. Biostimulation (enhancing the activity of native microbes); bioaugmentation (increasing the viable microbial counts); bioaccumulation (storage of toxic or nontoxic elements by the microbes) [29,30]; biosorption (removal of elements from the environment through adsorption) [31] where microbial transformation of metal ions involve the action of specific enzymes for oxidation, reduction, methylation, dealkylation and precipitation [32] and the use of biofilms [4] are some of the examples of recent advancements in microbial bioremediation techniques.
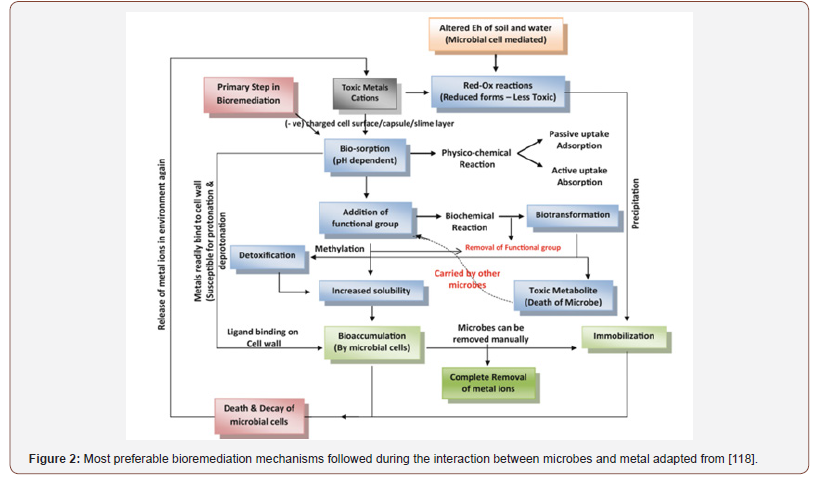
Higher microbial counts and diversity is directly associated with the higher content of organic matter in soil [33]. Generally, organisms rely upon associations with their neighbours for the sustenance of life [34]. Microbes also interact with other organisms within their surroundings for organic carbon, energy and shelter to metabolize, detoxify and accumulate metals mostly in cell wall like any other nutrient element. Microbes releasing chelating agents and acids altering physicochemical properties such as redox potential (Eh) of surroundings [35] bring substantial changes by increasing the bioavailability of metal ions [36]. Microbes have diversified conditions for their growth with ability to adapt to the changing environmental conditions [37]. Advancements in microbial remediation techniques and studies on plasmidencoded biochemical information and genetic engineering helped in designing new strains of known bacterial species for targeted bioremediation, e.g., recombinant Escherichia coli expressing metallothionein gene (Neurospora crasa) for cadmiun uptake, which was more rapid than the gene donor microbial species [38]. First step in the interaction between microbial cell and metal ions [32] followed by the physical adsorption, ion exchange and complexation, (Figure 2). Microbes have inherent ability of first rate to get adapted to the changing environment though they have certain limitations. In-depth understanding of microbial ecology is required for the improvement in the microbiological action and to predict the successful bioremediation process [25]. Figure 3 shows the environmental challenges that microbes face in their life.
Three types of factors affecting the microbial processes
• Physicochemical characteristics of environment or the abiotic factors,
• Biological factors or biotic factors and
• Climatic conditions whereby physicochemical and climatic conditions are among the major factors affecting the metabolic rates in microbes.
Materials and Methods
Study site
The study was conducted in the permanent military shooting/ training range located at 5 km east of Kachia town in Kaduna state, north central Nigeria. The shooting range was established in 1965 and it covers an area of about 24.95 square km that lies between longitudes 90 55’ N and 70 58’ E, with an elevation of 732 m above sea level and the topography is undulating and the vegetation is guinea savannah, Nigeria ecology. The area where the munitions/ explosive are fired (the impact area) is a valley consisting of about four large rocks, where the fired munitions/explosives land and explode during military training. Five military exercises involving the deployment of explosives are carried out annually by the Nigerian Defence Academy (NDA) Kaduna, Nigerian Air force (NAF) Kaduna, Nigerian Army School of Infantry (NASI) Jaji, Armed Forces Command and Staff College (AFCSC) Jaji and Nigerian Army School of Artillery (NASA) Kachia, Kaduna, Nigeria, (Figure 5).
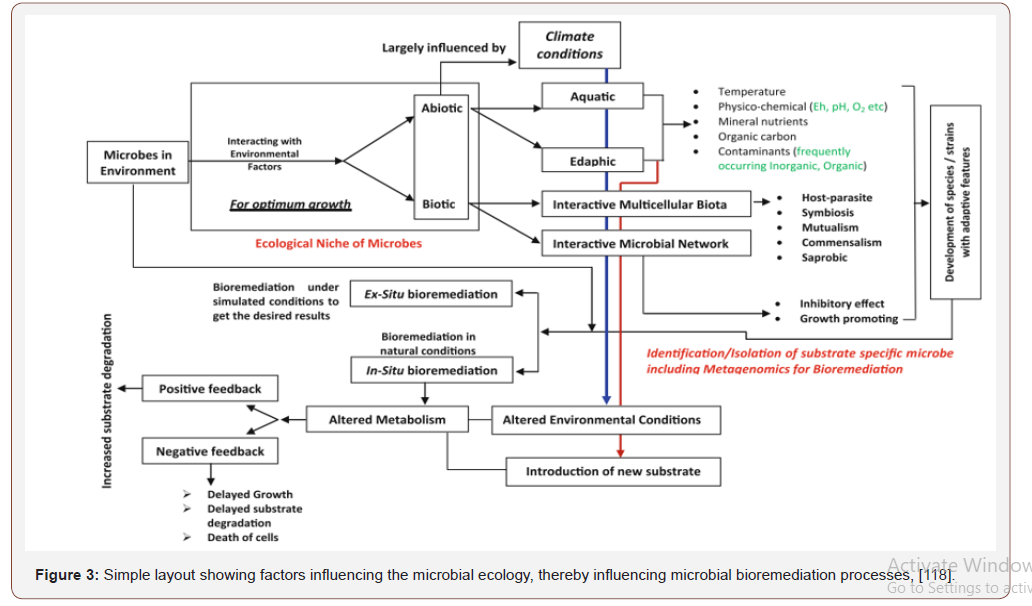
Sampling points
Four sampling points selected for the study are locations 1, 2, 3 and 4, Table 1. Locations 1 and 2 are the twin smallest rocks closest to the road. While locations 3 and 4 are much larger rocks heavily impacted by munitions/explosives between locations 1 and 2 is a flat ground where rain run offs flow through to the stream in the study area (map with global positioning system [GPS] co-ordinates). Locations 1 and 2 approximately 200m from the Plateau top, where the small arms are fired such as Kalashinokov, FN, Greenade, GPMG, SMG and Pistols. The soil in locations 1 and 2 is made of 50% silt and a flat ground with shrubs and drainage that flow through to the farmlands near the sites. Location 3 is approximately 9000m away from the Plateau top lies between 90 53’ 44.71” Northings and 70 53’ 17.87” Eastings. The impact area of locations 3 and 4 are mainly largely rocks containing high concentration of explosives due to the extensive use of bombardment by the artillery weapons, 155mm nortwizer, and other heavy weapons while location 4 is ahead of location 3 is about 10,000m from the Plateau top where heavy weapons are fired. The distance between locations 3 and 4 was covered with various shrubs and two major streams, Figure 4, Plates 1 and 2, respectively.
Sampling technique and Soil treatments
Soil sampling: Sampling was done during both dry and wet season, (Table 6 in Appendix 2). Four locations located within NASA shooting/training range Kachia were earmarked as sampling sites for this study using soil iron auger.10 gram of soil sample (0-30 cm in depth) with diameter of 9 cm were collected from 3 different points within a location and harmonized to form a composite sample at various locations of the sites. All sample collected were sieved using a 63 (106m) mesh size laboratory sieve and then stored in black labelled polythene bags until for analyses. Samples for microbial analyses Table 3 were kept in a cool box refrigerated with ice pack to retain the original microbial activities (Table 5 in Appendix 1).
Soil sample pre-treatment: Sampling points were treated in the laboratory before digestion commence. 10 grams of the soil sample was weighed into a clean dried beaker and put into an oven at about 100°C for one hour. The soil sample was then ground in a porcelain mortar with pestle and sieved through 250 μg mesh size to obtain a homogenous sample.
The soil sample was stored in sterilized polyellylere bags, label and kept for next stage of pre-treatment. This procedure was repeated for all the collected soil samples. The ground soil samples were used for analyzing heavy metal and explosives content for soil samples [39].
Table 1: Global positioning system (GPS) recorded in degrees, minutes and seconds (DMS) of sampling locations..
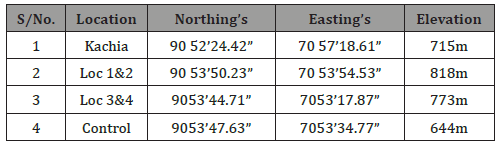
Digestion of soil sample for heavy metals and explosives analysis: 1 g of pre-treated soil sample was accurately weighed and placed in a 100 cm3 beaker. Then, 4 cm3 of 70% of HCLO4 and 2 cm3 of concentrated HNO3 were added to the sample and the resultant mixture was put in the oven at 100 0C overnight. A white ash was formed which was dissolved in 2 cm3 of 1m HCL the digest was filtered into 50 cm3 standard volumetric flask. The beaker was then rinsed with small portions of double distilled water and quantitatively transferred into the flask. The resultant solution was made up to the mark with double distilled water, transferred into a clear dried plastic sample bottle, labelled and kept for heavy metal and explosives analysis. This procedure was repeated for all the samples collected at this site. A blank was prepared using the same procedure excluding the soil sample.
Heavy metals analysis principle
The analysis was done using Atomic Absorption Spectrophotometer (AAS) at the laboratory of Geological Survey Kakuri- Schimadzu AAG50 model [40]. The digested soil samples were analyzed for heavy metals. Heavy metals analyzed include arsenic, boron, cadmium, chromium, cobalt, copper, iron, lead, magnesium manganese, nickel and zinc using AAS, according to APHA (1998) [41]. The technique makes use of absorption spectrometry to assess the concentration of analyzed in a sample by measuring the absorbance against a known concentration. It relies on the Bear Lambert Law.
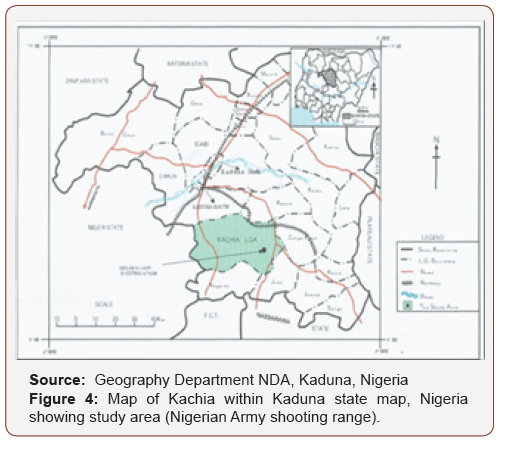
Explosive analysis
The analysis was carried out at National Research Institute for Chemical Technology (NARICT), Zaria, Nigeria. The vials containing the AcN (Acetonitrite) soil extracts were placed into Gas Chromatography (GC) auto sampler trays that were continuously refrigerated by circulating 0°C glycol/water through the trays, the extracts were analyzed by GC using a micro-electron capture detector (GC-μECD). Results were obtained on HP-6890 GC equipped with a micro cell Ni63 detector at 280°C according to the general procedure outlined, in EPA SW-846 method 8095 [42]. Direct injection μL of soil extract was made into a purged packed inlet port, at 250°C, that was equipped with a deactivated Restek Uniiner. Primary analysis was conducted on a 6 m x 0.32 mm ID-fused - silica column, with a 1.5-um film thickness of 5% (Phenyc) - methyl - siloxane (RTX -5 from Restek). The GC oven was temperature programmed as follows; 100°C for two minutes, 10°C minutes ramp to 260°C, twominute hold. The carrier gas was helium at 10 ml/minute (linear velocity approximately 90 cm/second). The ECD make-up gas was nitrogen flowing at 40 ml/minute. If a peak was observed in the retention window for a specific signature compound, the extract was reanalyzed on a confirmation column, 6 mx 0.53 mm ID having a 0.1 mm film thickness of 50% cyanopropylmethyl - 50% phenylmethylpolysiloxane (RTX - 225 from Restek). If analyze concentrations were taken from the determination on the primary column, unless they appear to be coalition with another compound, in, such cases reported concentration were taken from the determination of the confirmation column.
Screening for explosive/munitions degrading organisms
Nutrient Agar: Nutrient Agar (Antec/USA) by dissolving 28g of the Agar in one litre of distilled water in a conical flask. The conical flaks with the media was auto daved at 121°C at 15 Pressure Per Square (PSI) for 15 minutes. Cooled to 400C before pouring or dispensing if a sterile petri dishes.
Mackonkey Agar: Mackonkey Agar was prepared by dissolving 49.53g of dehydrated medium in 1000ml distilled water in a conical flask. It was heated to dissolve the medium completely. The medium was sterilized by autoclaving at 15 1bs pressure (121°C) for 15 minutes. Cooled to 45-50°C, mixed well before pouring into sterile petri plates.
Potato Dextrote Agar (PDA): Thirty-nine (39)g of dehydrated medium suspended in 1000 ml distilled water was heated to dissolve the medium completely. It was then sterilized by autoclaving at 15 1bs pressure (121°C) for 16 minutes. Cooled ten Percent (10%) of tartanic acid was added and mixed well to achieve pH 3.5 before dispensing into petri plates. The media were spread with the specimen soon after solidification of the media. Plates were incubated at 25-30°C in an inverted position (agar slide up) with increased humidity. Cultures were examined weekly for fungal growth and were held for 4-6 weeks.
Basal Salts Medium (BSM): Composition of what is BSA medium for, isolation and growth of bacteria degrading explosives. The ingredients in the order listed were added to 800 ml of tap water while stirring. Until the last ingredient was dissolved completely. The final medium was filtered through fine filter papers. Agar (2%) was added and sterilized in an autoclave at 121°C and 15 pressure per square (PSI) inch for 15 minutes. The normal pH is 6.9 to 7.0. Bacteria microbes like Bacillus spp have efficient biodegradative patways for xenobiotics [43].
Isolation of possible explosives degrading bacteria
Thirty (30) gram samples of soil from selected positive screening tests was placed into flasks and amended with one percent (1%) co-metabolite (sodium acetate) and 5 ml of distilled water.
Flasks were plugged and incubate at 28°C. After one week of incubation samples of the acetate primed sterile water were plated on to basal salts medium supplemented with 15g/l yeast extract cannot solidify medium nutrient agar and the co-metabolite at a concentration of 20g/l and 60 g/ml nystatin to suppress fungal growth. Plates were incubated at 28°C for two weeks. Representative colonies were picked from each plate and transferred to liquid culture media containing 100 mg/L TNT and incubated for 10 days at 28°C on a gyrorotary shaker at 150 rpm. Bacteria Isolates were identified by Blog identification (7 series of biochemical tests in liquid for metabolic characterization) and confirmed by reference to Bergey’s manual of systematic bacteriology.
Serial dilution: The sterile dilution blanks were marked in the following manner. 100 ml dilution blank was 102 and 9 ml tubes sequentially were 103, 104,10-6. One gram of soil sample was weighed from each sample located and added to the 12-2 dilution blank and vigorously shaken for at least one full minute with the cap securely tightened. All the 10-2 dilution was allowed to sit for a short period. The one ml from this dilution was aseptically transferred to the 10-3 dilution was again transferred to the 10-4 dilution. The procedure was done to 10-5 and 10-6. A flask of nutrient agar from the 45° C water bath was especially pounded into each petri plates for that set. 15 ml was poured enough to cover the bottom of the plate and mixed with the one ml inoculum in the plate. Each set was gently swirled on the bench so that the inoculum gets thoroughly mixed with the ager. All the plates were allowed to stand without moving so that the agar solified and set completely. The plates were inverted and stacked into pipette carnisters and placed in the incubator or at room temperature until after 48 hours the same procedures were applied for MacKonkey agar and PDA.
Isolation of possible heavy metals tolerant bacteria
The soil samples solution were inoculated into nutrient agar and mackonkey agar plates containing different concentrations of the heavy metals in their salt form such as Lead chromate (vi) (PbCrO4), Nickel chloride (NiCl3), Cadmium nitrate (CdNO3). Zinc sulphate (ZnSO4), Manganese (iv) Oxide (MnO2), Cobalt aluminates (CoAl2O3), Chromium chloride (CrCl3), Iron arsenosulphide (FeAs5) and Arsenic Trichloride (AsC3). The plates were incubated at 30°C for 24-48 hours. The bacteria were isolated and subculture into nutrient agar plates to obtain pure culture of each tolerant strain.
Pure culture of bacteria
Streak method was used to subculture the bacteria isolate from basal salt medium (BSM) with 15g/l agar containing 100 mg/l TNT and co-metabolite at a concentration of 20 g/l. An inoculating loop was used to pick each colony gently from the Basal salt agar plates. The colony was re-streaked gently onto the surface of a fresh nutrient agar plate. The bacteria plates (Table 3) were incubated at room temperature on a laboratory bench [44].
Characterization of bacterial isolates gram staining: Thin smear of colony from the petri dish containing the bacterial culture was prepared. The smears were air dried and heat fixed using slide rack. The smears were stained with crystal violet for 30 seconds, each slide was washed with distilled water for a few seconds using wash bottles and then each smear was treated with gram’s iodine solution for 60 seconds. The iodine solution washed off with 95% ethyl alcohol. Ethyl alcohol was added drop by drop, until no more colour flows from the smears. The gram positive bacteria were not affected while all gram negative bacteria were completely decolorized. The slides were washed with distilled water, allowed to be drained and treated with safranin smears for 30 seconds and was washed with distilled water and blot dry with absorbent paper and the stained slides was allowed to air dry. The bacterial that retained the primary stain (crystal violet) were gram positive and those that do not retain the primary stain were gram-negative, [44]. The slides were examined microscopically using oil immersion objective. Bacterial colonies were identified by gram’s staining and biochemical characterization according to Bargy’s Manual.
morphology of bacteria isolates: Isolated colonies of each bacteria were absorbed and culturally morphologically based on their superficial forms (circular, filamentous and irregular) elevation (flat, convex and umbonate), Margin (Lobate, convex or irregular), and shape spiral, rod or cocci using hand magnifying lens.
Biochemical characterization of bacteria catalase test principle
The enzyme catalase is present in some bacteria which breakdown hydrogen peroxide to water and oxygen. The principle was used for the detection of catalase enzyme in a bacterial isolated. Few colonies of the test organism were picked with a platinum loop from nutrient and Mackonkey Agar as the case may be and then applied in a drop of 10% hydrogen peroxide on a clean sterile slide. The production of gas bubble from the culture indicated positive reaction.
Citrate test: The simmons create agar medium contains the pH indicator bronollymol blue and medium citrate as the sole sources of carbon. Citrate is an enzyme found in some bacteria. Citrate is breakdown by citrate to oxaloactic acid and acetic acid. The breakdown is indicated in the medium by the change of colour from green to blue. However, the removal of citrate from the medium by bacteria that can utilize citrate creates an alkaline condition in the medium and indicator changes to its alkaline colour blue. The test organism were inoculated into simmon’s citrate agar slants and incubated at room temperature for 24 hours. Colour change from green to blue indicated a positive test while no colour change indicated a negative result.
Coagulase test: Coagulase is an enzyme aureus in staphylococci aureus that causes the conversion of soluble fibrinogen into insoluble fibrin. The reaction is different from the normal clothing mechanism exhibited in blood clothing. Gram positive staphylococci that produced catalase positive reaction were further tested for coagulase. 0.5ml suspension of a 24 hours broth culture of the bacteria was mixed with 1ml of anticoagulant containing blood plasma in a test tube. This was incubated at room temperature and positive coagulase bacteria revealed the formation of fibrin cloths in the test-tubes. Positive coagulase is a characteristic of staphylococcus aurens aura [45].
Mortality indole orinthine agar
Mortility can be determined from Brownian motion (small, random movement) exhibited by the bacteria in the medium. Trptophanase is oxidized by an enzyme trypotophanase in some bacteria to produce indole pyruvate react with Kovaes, reagent (dimellhycamino-benzaldehyde) to form resounded (cherry red compound) and water. Bacteria with Ornithine decarboxylase will decorboxylate the amino acideornithine into purescine. The medium which contains bromcyresol purple with turn yellow after the enzyme inedecarboxylateornithine.The amino acid ornithine into putrescine. The medium which contains bomcresol purple will turn yellow after the enzymedecarboxylate ornithine. Mortility indole ornithine (M10) medium was used to determine motility and indole production. Ten (10) ml of the medium was dispensed into a test-tube and autoclaved. Each pure colony of the unidentified organism was stabbed into the test tube using a sterilized wire loop and incubated at room temperature for 24 hours. Motility was observed as hazy diffuse spreading growth (Swarm) along the stab unlike non motles, restricted to the stab line. The presence of ornithine decarboxylase is observed when the purple colour of the medium changes to yellow [46]. For indole production 2-3 drops of Kovac’s reagents (Dimellylamino-benzylaldehyde) was added to the medium in the test tube. A red precipitate at the top of the interface indicated a positive reaction of indole production. For Ornithine decarboxylase reaction [47].
Methyl red test: Some bacteria produces large amount of organic acids such as formic, lactic acid and succinic acid. These organic acids react with methyl red resulting in red coloration of the medium. The test was conducted to detect the production of sufficient acid by fermentation of glucose so that pH dropped to 4.5. The test organism were inoculated in glucose phosphate broth and incubated at room temperature for 2-5 days. Five drops of 0.04% solution of methyl red were added and mixed well. A bright red colour indicated a positive result while a yellow colour indicated a negative result.
Oxidase test: Certain bacteria contain a terminal cytchrome C and its associate oxidase found in their respiratory chain. Bacteria which contains such a chain can oxidize chemicals such as Kovac’s Oxidase reagent (1% tetramethy l-p-phencle-nediamine dihydro chloride). Electrons are transferred from the chloride). Electrons are transferred from the reagent to cytochrome and hence, through the oxidase to oxygen. When oxidized, the reagent develops an intense violet colour. A drop of 1% solution of oxidase reagent was added on a piece of filter paper. Few colonies of the test organism were rubbed onto it. The production of a deep purple colour within 10 seconds indicated a possible reaction.
TSI test: Triple sugar iron agar contains glucose, lactose, sucrose, sodium thiosulphate and a ferrous. The fermentation of any of the sugar results in a yellow colorations of the medium butt or slant. Hydrogen sulphide is produced from the reduction of amino acids such as cysteine and methiomine. Hydrogen sulphide when produced reacts with the metal salt (ferrous sulphide) to form a visible black. Ferrous sulphide precipitates. If gas is produced, this would give risk to crack in the butt of the medium due to the production of oxygen from the organism stab into the butt of the medium. Agar slants were prepared in test-tubes. Each pure colony of the unidentified bacteria was picked with a sterilized wire loop and stabbed into the butt of the test tub. Colonies were serpetinely streaked on the slope before stabbing into each butt and allowed to uncubate at 30°C for 24 hours. When only glucose was fermented, the slant was red (alkaline) and butt turned yellow. If lactose or sucrose was fermented as much acid was produced that both the slant and butt remained yellow (acid). Gas production resulted in a cracks or the medium was pushed upward. H2S production was confirmed by the change of the medium colour to black along the streak line.
Nitrate reduction: The nitrate test is used to determine bacterium that can reduce nitrate. The medium consist of potassium nitrate which when reduced turn the medium from yellow to red. The test organism was inoculated in 5 ml medium containing potassium nitrate, popatone and distilled water which were incubated at 200m temperature for 96 hours. 0.1 ml test regent which consist of equal volumes of 0.8% sulphanilic acid and 0.5% naphylamine in 5N acetic acid was mixed before use. A red colour developing of nitrate hence the ability of the test organism to reduce nitrate to nitrate.
Urease test: The urea medium contains the pH indicator phenol red and urea. Urea is a major organic waste product of protein digestion in most bacteria. Urease is a hydrolytic enzyme in some bacteria which catalyze urea into carbon dioxide and ammonia. The ammonia liberated would dissolve to create an alkaline medium and the indicator would change to its alkaline colour red. The ability of an organism to produce urease enzyme was evaluated by this test: The test organism were separately inoculated on the entire slope of Christensen’s medium which contains urea and phenol and indicator. It was incubated at room temperature and examined after 4 hours overnight incubation development of red colour indicated production of urea enzymes by test organism.
Isolates of explosive degrading bacteria from kachia firing range
Eight sets of 250 ml flasks were prepared for the work. The nutrient broth was sterilized in an autoclave at 1210 for 15 minutes.120 ml of nutrient broth was incorporated with tenmillimeter mineral salt and 1% explosive concentration into each of the nine flasks. The first flask was left as blank while second, third, fourth, fifth; sixth, seventh and eighth flasks were inoculated with each of the isolated bacteria. The ninth flask was inoculated with a mixed culture consortium of the seven bacteria isolates. It was incubated for 14 days at room temperature. The growth of the bacteria was measured by taking the optical density (O.D) using a spectrophotometer at 600 nm from 0 hour to 14 days at regular interval of 2 days against a blank containing broth with 1% explosive concentration [48].
Bacteria isolates to various concentrations of some heavy metals
The isolated bacteria were each inoculated into a nutrient broth containing different concentration of heavy metal, 0.5mg/ml, 1mg/ ml, 2 mg/ml, 4 mg/ml, 6 mg/ml and 8 mg/ml respectively for Pb, Ni, Cd, Zn, Mn, Co, Cr, Cu and As. The experiment was left for 24 hours and the inhibitory concentrations were measured using a spectrophotometer at an absorbance of 600nm against a nutrient broth (blank) containing the same amount of heavy metals.
Isolation of genomic DNA from bacteria
Bacteria with proven bioremediation capacity (also efficient strains of these bacteria) was selected and the DNA isolated. DNA of bacteria strains isolated was extracted from 1ml of bacteria culture, the culture was pelleted by centrifuging at 12,000 rpm for 2 minutes, the pellet was treated with lyses solution and proteinase K and incubated at 600C for 30 minutes. Nucleic acid were precipitated with isopropanol by centrifuging at 10,000 rpm for 10 minutes, washed with 1ml of a 70% (v/v) ethanol solution and dissolved in 0.1ml of a TE buffer (Figures 5&6). The purity and quantity of DNA were examined by recording its agarosegel electrophoresis [48,49].
Bioremediation of heavy metals in soil
The soil samples solution was sterilized using an autoclave at 120°C for 15 minutes. 100 ml of the sterilized soil solution was poured into fifteen conical flasks. The first flask was not inoculated with bacteria (control), the second, third, fourth, fifth, sixth, seventh, eighth, ninth, tenth, eleventh, twelfth and thirteenth flasks were each inoculated with Acbrombacter spp; Bacillus subtilis, Lysinibacillus spp, Arcobacter spp, E. coli, Enterobacter spp and Escherichia spp respectively. The experiment was left to stand for 24 hours after which the concentration of Cadmium, Chromium, Nickel, Zinc, Cobalt, Iron, Lead, Cupper and Arsenic were measured using Atomic Absorption Spectrophotometer (AAS).
Bioremediation of explosive in soil
Nutrient broth and soil solution were added in a ratio 4:1.8 set of 250 ml flask were set for the work. The broth and soil solution were sterilized in an autoclave at 121°C for 15 minutes. 120 ml of nutrient broth and 30 ml of sterilized solid solution were added into each of the fifteen flasks. The first flask was left uninoculated, but the second flask up to the eleventh flasks was inoculated with the isolated bacteria and fungi from explosive/munitions which are Achromobacter spp., Bacillus subtilis. Lysinibacillus spp., Enterobacter spp, Escherichia coli. The experiment set-up was left to stand for 14 days and the micro remediation was measured by taking the optical density (O.D) reading at 595 minutes from 0 hours-14 days at regular intervals of 2 days against the control [50], Figures 6&7.
Polymerase chain reaction amplification of 16srRNA Gene
The PCR reaction mixture containing 10X PCR buffer, 25 mm, magnesium chloride, 25 mm dNTP’s, 10pm/ul Primer concentrations and template DNA were used for the amplification of the 16srRNA gene for each isolates. PCR conditions were optimized using lab net thermal cycler. The PCR program began with an initial 5-min denaturation step at 94°C: 35 cycles of 94°C for 45 seconds, annealing (1 minute at 55°C), 10 minutes extension step at 72°C. All reaction mix were preserved at 4°C until it was time for analysis as reported by Kloos in 2006. The amplified 16srRNA gene of each isolates was further characterized using gel electrophoresis.
Sequence determination of 16srRNA gene: The amplified 16srRNA gene of each isolate was processed for sequencing and characterization. The sequencing Kit (Applied Biosystems) with the product was analyzed with ABI prism DNA sequence (ABI). The gene sequence of each isolates obtained in this study were compared with known 16srRNA gene sequences in the Gene Bank database as described by [48].
Bacterial biodegradative activity by turbidometry: Eight sets of 250 ml flasks were set for the work. The broth was sterilized in an autoclave at 121°C for 15 minutes. 120 ml of nutrient broth was inoculated with 1% munitions/explosives concentration into each of the seven flasks. The first flask was left uninoculated with bacteria isolates but the second, third, fourth, fifth sixth and seventh were inoculated with the isolated bacteria which are Enterobacter, Escherichia, Klebsiella, Arcobacter, Bacillus, Lysinibacillus, Achromobacter spp respectively. The eight flask was inoculated with a mixed culture consortium of the seven bacteria and was measured by taking the Optical Density (O.D) readings at 595nm from 0 hours - 14 days at regular intervals of 2 days against mineral salt medium as blank [47].
Statistical analysis
Data collected from the study were analyzed using general descriptive statistics, one way Analysis of variance (ANOVA) at 95% probability level of significance. If significant differences were found. Duncan’s Multiple Range (DMR) test was used to compare the different experimental groups. Computer Software Statistical Package for Social Scientists (SPSS) and Microsoft-Excel were used for the statistical analysis.
Polymerase chain reaction (PCR) for amplification of catabolic genes
PCR consist of an exponential amplification of DNA fragment and the principle is based on the mechanism of DNA replication in vivo, double stranded DNA is denatured to single stranded DNA, each single strand DNA is anneal by the forward and reverse primers of known sequence and elongated using tag DNA polymerase to produce copies of DNA template. Isolated explosive bacteria DNA was amplified using catechol 2,3 dioxygenase gene primers. The genomic DNA, the primers and PCR master mix were added into a PCR tube. The tubes were spun to collect the droplets. The tubes were then inserted into the PCR machine while maintaining the regulation for initial denaturation (45 seconds at 94°C), annealing (1minute at 55°C) extension (1minute at 72°C) and final extension (10 minutes at 72°C). The amplified catabolic genes were resolved in 1.5% agarose gel electrophoresis stained with ethidium bromide and viewed under ultra-violet (UV light).

Results and Discussion
Physio-chemical properties of the military firing ranges
The study area 34.22°C dry season and 27.16°C wet season were recorded as an integral of the climate of the region; also within similar work by Jenkins [51] 27-35°C on characterization of explosives contamination at military firing ranges .The explosives values obtained within Federal Ministry of Environment, Nigeria (FMENV) limit of <40 μg kg-1 with soil pH of 6-8 value suitable microbial bioremediation [52]. The high value of electric conductivity EC recorded during the wet season (Table 4, Appendix 1) similar to finding of Oaikhena in 2015 and by Duniya [53] respectively. The value of (EC) is above the FMENV and WHO [54- 57] permitted limits of 500/μ5/cm. The low values of total dissolved solutes for both wet and dry season were [58] within the value of 2000 mg/l acceptable by FMENV. Which is contrary to the finding of Oaikhena in 2015, whose values were within 2400 - 5900 mg/l. and is in agreement with Usman [50] and Duniya [53] the high values of total dissolve solid (TDS) would be due to the deposit of organic and inorganic matters necessitated by the military activities, Figure 4. Xenobiotic analyzed during dry season are higher than those in wet season as indicators of the soil pollution detrimental to both human and the environment (Table 6, Appendix 2). The soil had low clay percentage which facilitates increase ventilation (Figure 4). Increased ventilation has a direct impact on microbial growth which can enhance the biodegradation of explosives. The water holding capacity of the contaminated soil samples under investigation was observed during this study (59.56) is in the ranger suggested for optimal bioremediation [52]. From the result in (Table 5 Appendix 2) it was observed that the concentration of heavy metals varies significantly (P>0.05) between the concentration of toxic metals in both dry and wet season samples which invariably will affects the bacteria biodegradation of the pollutants of concern as affirmed on TNT degrading bacteria.
Concentration of the toxic metals was also observed to be higher in the contaminated site than the uncontaminated site because of the activities of military activities affirmed by similar work. The average concentration of the metals was higher during the dry season than the wet season, as a results of metals washed as runoff into the drain as a result of rainfall, Table 6. Whereas, the lower level concentration of heavy metals found in the dry season could be attributed to high temperature which may have constantly account for increase and decrease in the rate of radiation in dry and wet season [59]. The result shows that lead (Pb) with (27.01) mg/ kg) in location 1 which was significantly higher (P>0.05) than other metal is probably a recalcitrant metal to micro-remediation (Table 6, Appendix 2). WHO has recommended >10μg/l lead as safe permissible level in the drinking water. In zero metre, TNT, RDX and HMX were consistently found at the highest values of 49.39 mg/kg, 68.19 mg/kg and 35.67 mg/kg, respectively.
An impact area and beyond within the hand grenade range, a 105 mm howitzer firing point and the heavy artillery and mortar range that had been analyzed by GC-ECD. TNT concentrations ranged from 0.11 to 49.39 mg/kg in dry season and 0.12 to 26.6 mg/kg in wet season. HMX concentrations ranged from 0.69 to 35.67 mg/kg in dry season and 1.43 to 28.21 during wet season, then RDX concentrations ranged from 0.49 to 68.19 mg/kg in dry season and 0.38 to 19.9 mg/kg during wet season, while, PETN concentration ranged from 0.39 to 4.38 mg/kg in dry season and 0.17 to 7.12 mg/kg during wet season similar to the report [50]. Among all the concentration of explosives both in dry and wet season RDX recorded the highest concentration of 68.19 mg/kg implies that the Kachia shooting range is polluted with explosives most especially the RDX and agreed by EPA according to Lachance [22].
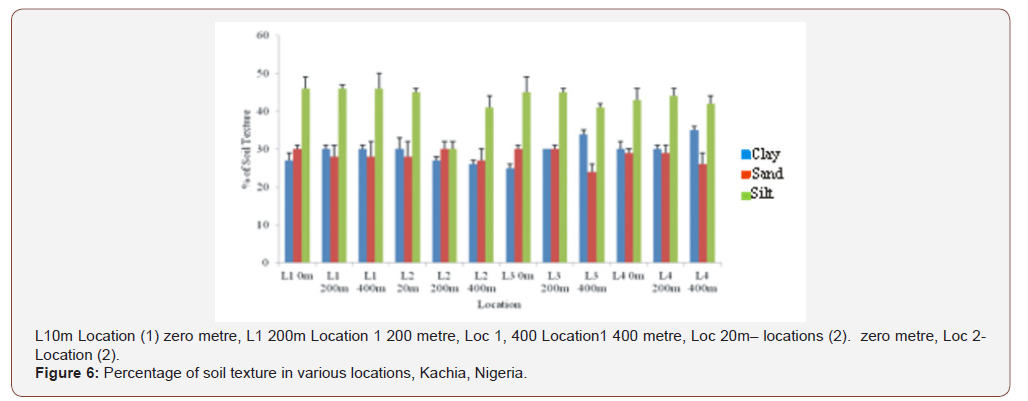
Bacteria Consortium Explosives Degradation Bacteria consortium biodegradation: Explosive by Turbidometry
Figure 7 shows the optical density reading of biodegrading activity of each bacterial isolate on explosives broth with 1% exposure mineral salt medium (MSM). The results revealed that the bacteria Lysinibacillus spp, Achromocter spp, E. coli, Klebsiella pneumonia, Bacillus subtilis, Arcobacter spp and Enterobacter spp, had the ability to degrade explosives. The result of analysis shows that there is a significant difference in the overall growth rate reading at 595 nm for 14 days of incubation, Figure 8. The overall results also indicated that growth rate increase significantly from the 8th to 14th day Achromobacter and Arcobacter had Lag, exponential, stationary and death phases. Lysinibacillus, E.coli and microbial bacteria consortium (MBC) growth rate observed was exponential, stationary and death phases while Bacillus subtilus, Klebsiella Pneumonia and Enterobacter spp. exhibited stationary, exponential and death phases. The test on the degrading activity of isolates on explosive show that bacteria genus Lysinibacillus, Achromobacters pp, E.coli, Klebsiella Pneumonia, Bacillus subtilis, Arcobacter spp, Enterobacter spp and MBC were potent degraders of explosive with MBC (Table 2) > Lysinibacillus > E.coli > Enterobacter spp > Bacillus subtilis > Klebsiella pneumomia > Achromobacter > Arcobacter spp with optical density values of 0.182, 0.118, 0.099, 0.097, 0.084, 0.080, 0.072 and 0.067 respectively . Growth of bacteria between 0-2 days was slow. Meanwhile, the number of bacterial species that had the peak in day 12 were Lysinibacillus, E. coli and Bacillus subtilis respectively (Figure 9). Anova test for total explosive contents shows a significant difference for all locations in both dry and wet Seasons (P<0.05) according to the Duncan multiple range test, (Table 6, Appendix 2).
Bacteria heavy metals biotransformation and mineralization
Bioremediating potentials of bacterial isolates and consortium and to heavy metals: In Figure 11 certain bacteria isolate with different percentage reduction of heavy metals was observed when compared with control and values obtained. Lead concentration was reduced by Lysinibacillus to 25.8%, Achromobacter spp to 12%, E. coli to 54.7%, Klebsiella pneumonia to 42.7%, Bacillus subtilis to 55.1%, Arcobacter spp to 43% Enterobacter spp to 65.5% and MBC to 75.4%. Cadmium was reduced by Lysinibacillus to 24.6%, Achromobacter spp to 24.86%, E.coli to 21% K. pneumonia to 15.3%, B. subtillis to 100%. Arcobacter spp to 13.14%, Enterobacter spp to 21.2% and MBC to 100%. Zinc was reduced by Lysinibacillus to 37.8%, Achromobacter spp to 31.5%, E. colito 38.1%, K. pneumonia to 35%, B. subtillis to 38%, Arcobacter spp to 30.5%, Enterobacter spp to 35.5% and MBC to 37.9%. Cobalt was reduced by L. bacillus to 30.4%, Achromobacter spp to 30.8%, E. coli to 29.6% K. pneumonia to 30% B. subtilis to 100%, Arcobacter spp to 30.3%, Enterobacter spp to 30.6 and MBC to 100% (Figures 11&12). Copper was reduced by L. bacillus to 29.8%, Achromobacter spp to 8.1%, E.coli to 22%, K. pneumonia to 27.6%, B. subtilis to 32.3%, Arcobacter spp to 2.1%, Entrobacter spp to 10.1% and MBC to 32.4%. Manganese was reduced by L. bacillus to 30%, Acromobacter spp to 16%, E.coli to 32%, K. pneumonia to 9%,B. subtilis to 18%, Arcobacter spp to 25%, Entrobacter spp to 34% and MBC to 51%. Nickel was reduced by L. bacillus to 22.21%, Achromobacter spp to 28.19%, E. coli to 14.1%, K. pneumonia to 19.1% B. subtilis to 22.6%, Arcobacter spp to 21.19%, Enterobacter spp to 14.9% and MBC to 38.3%.
Table 2: The growth rate (optical density values) of microbial bacterial consortium..

Table 3: Gram Stain Result, Morphological and Biochemical Characterization of the different microbial Isolates from the NASA Shooting Range Kachia Kaduna..
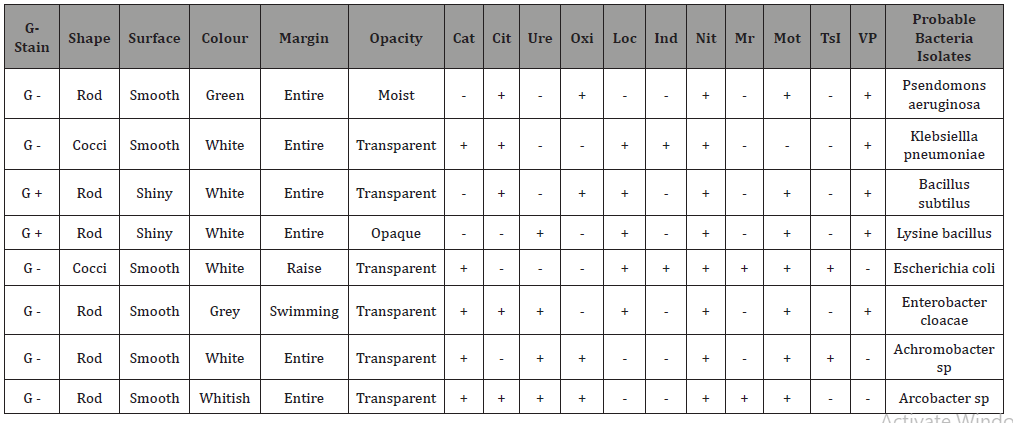
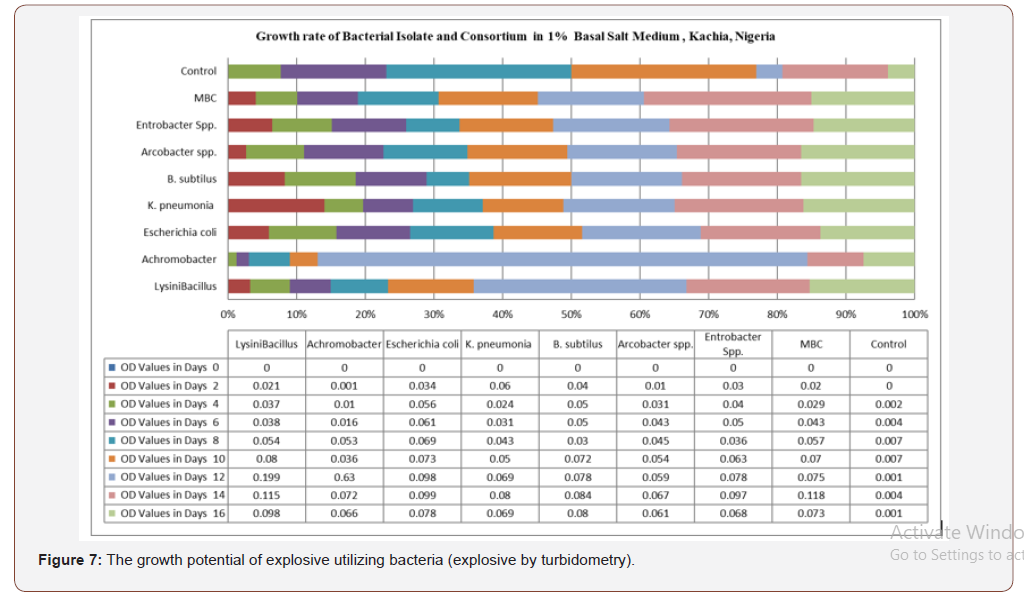
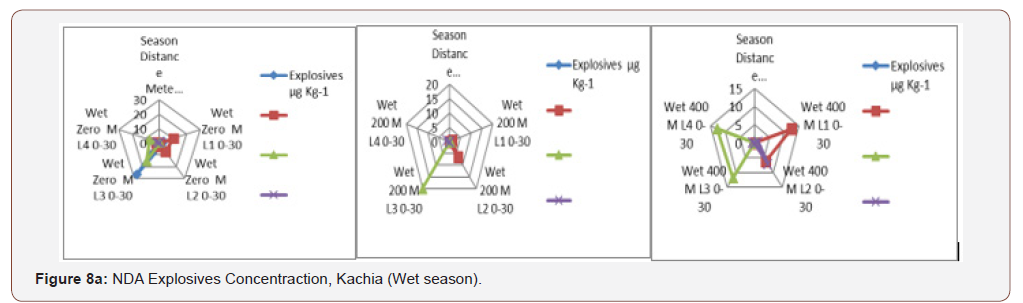
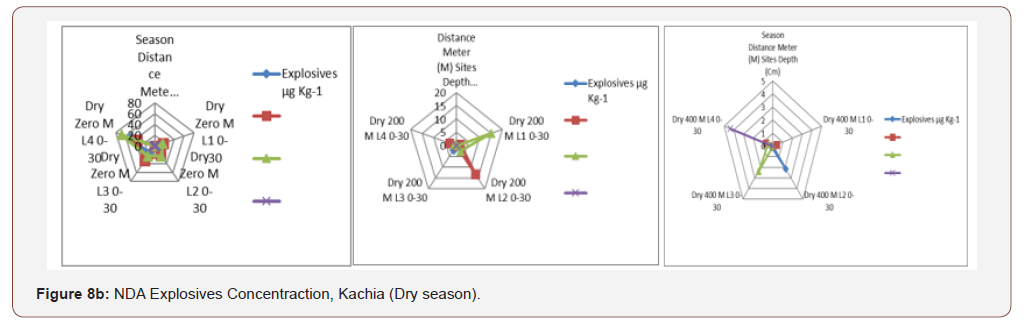
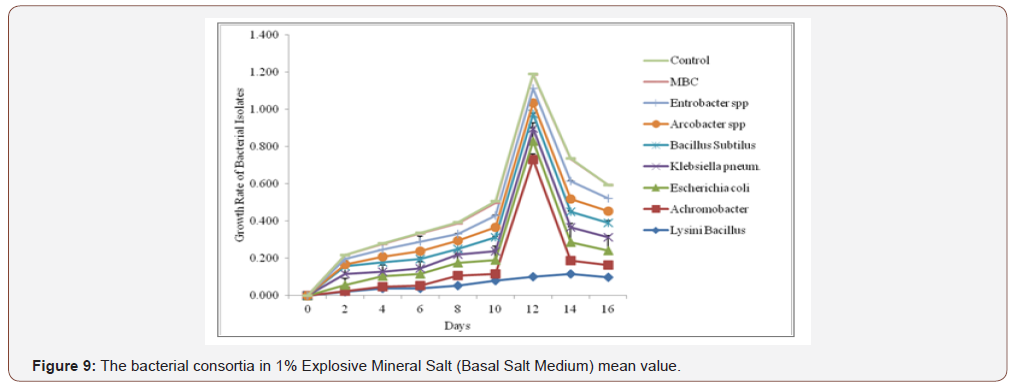
Chromium was reduced by L bacillus to 10.6%, Achromobacter spp to 20.7%, E. coli to 40.5%, K. pneumonia to 9.8%, B. subtilis to 45.3%, Arcobacter spp to 40.3%, enterobacter spp to 12% and MBC to 49.3%. Arsenic was reduced by L bacillus to 13.85%, Achromobacter to 6%, > E. coli to 11.1%, K.pneumonia 11% B. subtilis to 20.1%, Arcobacter spp to 12.9% Enterobacter spp to 5.96% and MBC to 20.5%. All bacteria isolate reduced heavy metals better than the control. The best isolates for heavy metal reduction in decreasing order were Bacillus subtilis>Enterobacter spp> E. coli >Arcobacter spp> Klebsiella Pneumonia > Lysinibacillus >Achromobacter spp. In the overall, the mixed culture consortium has the highest and prominent percentages reduction of each on every heavy metal analyzed in this study. Anova test for total explosive contents shows a significant difference for all locations in both dry and wet Seasons (P<0.05) according to Duncan multiple range test, Table 6, Appendix 2. Mixed bacterial consortium (MBC) observed high bioremediation potential (68.62%) compared to single isolate, Figure 8 whose bioremediation potential observed in decreasing order were Bacillus subtillis, Enterobacter spp, E. coli, Arcobacter spp, Klebsiella pneumonia, Lysini bacillus and Achromobacter spp in the following order as (55.1%), (54.80%), (54.1%), (43%) (42.7%), (37.8%) and, (31.5%) respectively. MBC showed high percentage degradation of explosive which might be attributed to the synergistic effect between the catabolic enzymes in the eight bacteria isolates. These findings are similar to the result obtained by Duniya [53] whose research reported biodegradation rates at 97% by MBC.
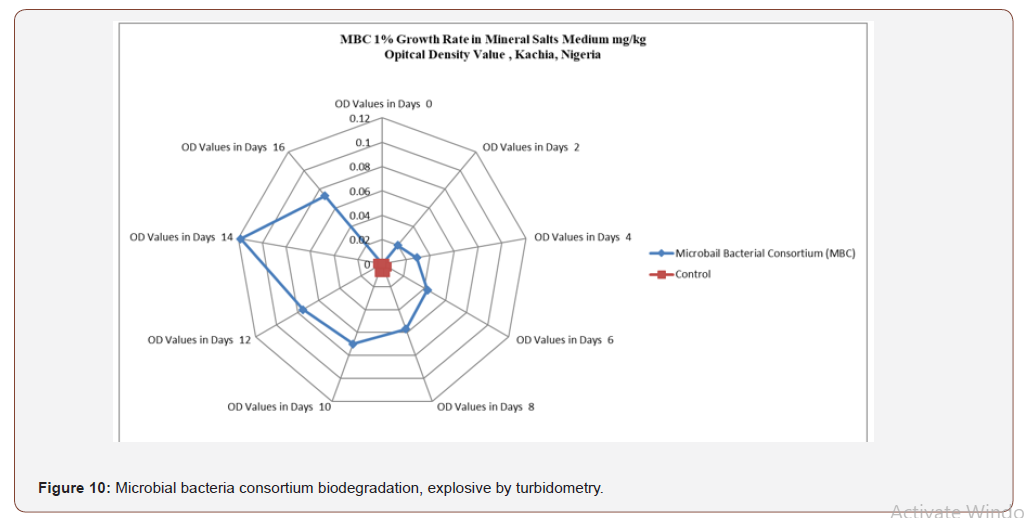
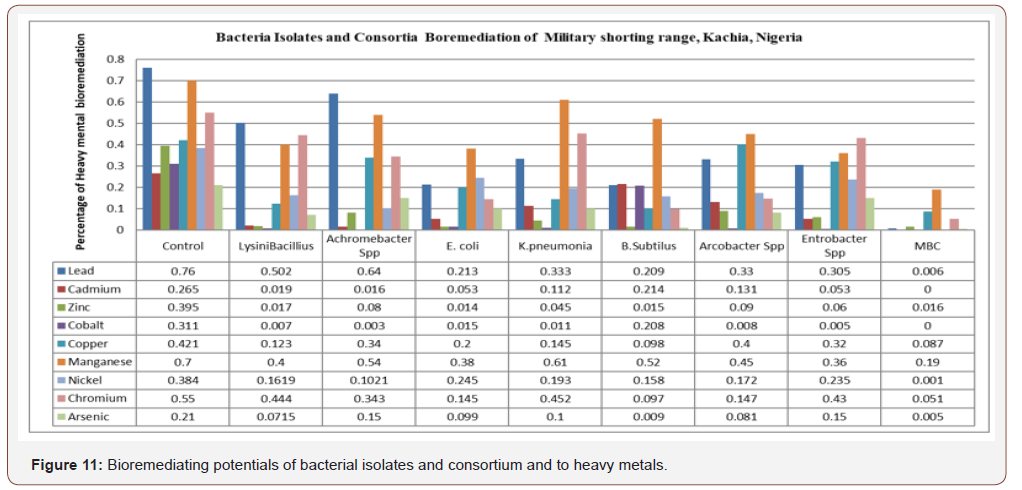
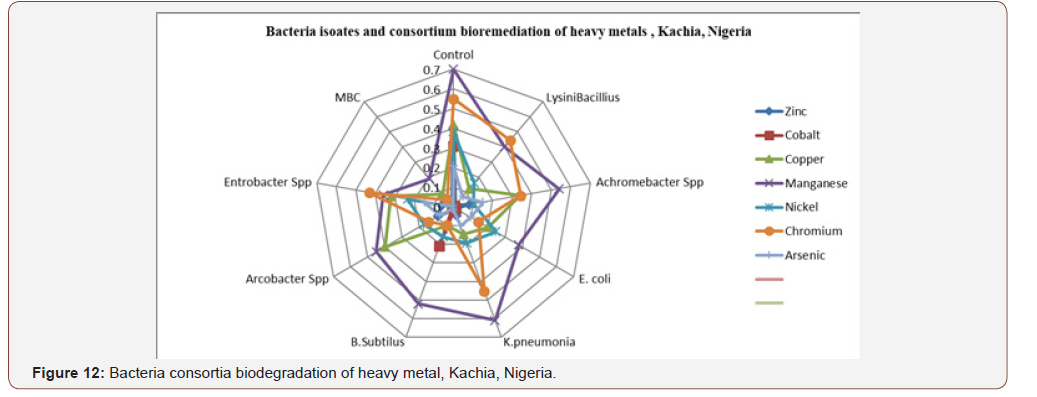
In these results, Figures 11&12 Achromobacter spp and Acrobacter spp experienced lag, exponential, stationary and death phases which are main four phases of growth contradict with the finding of Ojima in 2004, by Usman [53] in 2012 and Oaikhena in 2015 who reports that bacteria isolates did not exhibit lag phase of growth. Lysinibacillus, E.coli and MBC growth rate observed was exponential, stationary and death phase while Bacillus subtilis, Klebsiella Pneumonia and Entcrobacter spp exhibited stationary, exponential and death phases, result similar to Usman [53] in 2012, reported and similar to Figure 12 result. The efficacy of a desired insitu catalytic activity (biodegradation) depends first on its presence in the target site. One key enzyme may not be there, or it may preexist in the site but not be manifested. Alternatively, it can be hosted by just a very minor part of the whole microbial population, so that its factual expression in the site might not be significant [60] and allows for bio augmentation microbes inclusion for bioremediation.
Significance of microbial bacteria consortium
Generally, organisms rely upon associations with their neighbours for the sustenance of life [34]. Microbes also interact with other organisms within their surroundings for organic carbon, energy and shelter to metabolize, detoxify and accumulate metals mostly in cell wall like any other nutrient element. Mixed microbial communities (Figure 9) have the most powerful biodegradative potential because the genetic information of more than one organism is necessary to degrade the complex mixtures of organic compounds present in contaminated areas as reported by Fritsche [61] explained the impacts of MBC of the site xenobiotics.
Microbes releasing chelating agents and acids altering physicochemical properties (Table 4, Figure 13, Appendix 1) such as redox potential (Eh) of surroundings [35] bring substantial changes by increasing the bioavailability of metal ions [36], Figure 4. Higher microbial counts and diversity is directly associated with the higher content of organic matter in soil [33] narrated in Table 5, Appendix 1.The consequence is the application of biosimulants on the treated and post treated xenobiotics site as reported by Muter in 2012 biostimulate the capacity of bacteria to degrade TNT because several inherent characters of microbes that affect the substrate degradation [62]. Xenobiotics requires complex multispecies interactive networks for biodegradability and reported in similar work by Pandey and Jain [63] with plasmid-encoded genes provide specificity for substrates because organohalide respiratory bacteria thrive only in consortia, and their isolation and culture is very difficult. [64] reported that the optimum growth conditions of microbes are unpredictable. Initiation of microbial degradation of xenobiotics depends on the toxic pressure exerted by the contaminants on the microbes that induce enzymatic modifications [63], Figure 14, Appendix 3.
Klebsiella pneumomia bacteria inoculum degrade TNT by up to 90 % after 14 days incubation as reported by Muter in 2012 this is a function of Nitroreductases which are ubiquitous in bacteria and localized in the cytoplasm where they are constitutively expressed as Type I nitroreductases. This is expressed in gramnegative bacteria, such as Escherichia coli [65], Enterobacter cloacae [66,67], Klebsiella spp. Kim and Song [16] they catalyse the pyridine nucleotide dependent reduction of nitro-aromatic compounds which are related to the old yellow enzyme (OYE) of yeast [16,68,69]. Gram-negative bacteria are the best candidates for the microbial incorporation in self-cleaning explosive formulations since they are more tolerant to TNT than Gram-positive bacteria [70].
Escherichia coli bacteria has been genetic engineering on plasmid-encoded biochemical information recombinant expressing metallothionein gene for improved Cd uptake in reports by Pazirandeh [38] and abHan Han [32] which could have implications for cadmium reduced by E.coli to 21% compared to MBC to 100%, Figure 11 biodegradation expressed in the interaction between microbial cell and metal ions,as reported by Han and Gu, 2010. Several studies have reported the anaerobic degradation of RDX and HMX by pure bacterial cultures failed in aerobic condition except anaerobic condition under a microbial consortium like Enterobacteriaceae, Citrobocter freundii. Bacteria may possess reduction mechanisms that are not coupled to respiration, but instead are thought to impart metal resistance. For example, reduction of Cr (VI) to Cr (III) under aerobic condition [71].
Microbial competition and biodegradability
Stepwise denitration of RDX involves a nitrate reductase which is a ubiquitous enzyme possessed by a diverse group of bacteria, especially denitrifying bacteria [72] which affirm the role of bacteria consortium during bioremediation. Degradation of RDX through denitration and ring-cleavage involves the microbial P450 system which was shown to be able to degrade RDX under both aerobic and anaerobic conditions [73]. The main degradation pathways described involve either a sequential biotic reduction of the nitro functional groups followed by abiotic ring-cleavage [74] or a direct denitration followed by hydration and subsequent ring-cleavage [73] also reported the role of micobes consortia in bioremediation, (Figure 3&14).
Reduction of metals can occur through dissimilatory metal reduction [75], where bacteria utilize metals as terminal electron acceptors for anaerobic respiration are the mechanisms of bioremediation of the sites xenobiotics .Environments with a high nutrient complexity Figure 4, Plate 1 and Plate 2 respectively, containing multiple resources or niches, can reduce selection for competition [76], particularly if each species is limited by a different resource (Resource Ratio Theory) [77,78] explained in Figures 8 & 9 by Lysini bacillus, E.coli and MBC growth rate observed was exponential, stationary and death phase while Bacillus subtilis, Klebsiella Pneumonia and Entcrobacter spp exhibited stationary, exponential and death phases, result similar to Usman [50], that elaborated the microbial competition, Figure 14, Appendix 3.The two main resources necessary for microbial survival are nutrients and space from the pollutants site. Nutrients essential for microbial growth and metabolic functions include: carbon, nitrogen, phosphorus, sulphur, hydrogen, calcium, iron, and other metals [79-82]. A third and less commonly considered resource is genetic material. DNA is used as a nutrient source, but it may also provide its host with beneficial traits, enhancing its ability to survive and adapt [83].
Empirical data from this and other microbiome studies indicate that, in agreement with the ‘habitat filtering’ principle, species with similar resource requirements tend to live in similar areas of the body [84-86] which may explain local competition. Another possibility is that, as weaker strains are outcompeted in the soil, diversity is reduced. And because high diversity isolates competitors from each other through buffer zones [87,88] novel warfare may be enhanced between the remaining strains as the buffer zones disappear. [89] have shown using a theoretical model, that this can lead to ecologically stable equilibria wherein different species neutralise all produced antibiotics, and diversity is maintained. Apart from ecological conditions, different strains can remain mixed in space if they depend on each other for growth and survival [87,90-92]. The presence of neighbouring colonies also alters the competitive behaviour of many species of soil bacteria [76,93,94]. However, this may occur in a number of different ways as narrated in Figure 14, Appendix 3 xenobiotic biodegradation.
Three ecologically stable outcomes of competition are well accepted
• the less competitive strains go extinct while others dominate the community [93,94]
• strains continue to coexist by occupying different metabolic niches, where each specialises on a different resource type, or
• strains separate into different spatial niches or patches. Habitat filtering’ principle using Escherichia coli (species community member) can metabolically shift from fermentation to respiration when oxygen is present, generating high growth rates but low yield, allowing them to absorb nutrients faster than their competitors [97-100].
Of the best-studied systems involving the interplay between these two competitive mechanisms, cooperation that allows more access to nutrients, and cheating that saves the cost but relies on the presence of co-operators is the production of iron-chelating siderophores [101-105] and of quorum sensing (QS) molecules that coordinate the expression and production of exofactors [106,107] enzymes, rhamnolipids allow cells to swim to new areas or push competitors away [671,108] adhesins bind to surfaces and prevent displacement by invaders [69] extracellular polysaccharides (EPS) can smother and starve competitors, while also pushing clone-mates into nutrient-rich environments [109-111], E. coli cells produce surfactants and EPS that inhibit biofilm formation in Staphylococcus aureus and Pseudomonas aeruginosa [112,113]. Bacillus subtilis produces enzymes that degrade QS molecules in Vibrio cholerae, which is subsequently unable to form biofilms [112,114].
Conclusion
This study explained the munition synergistic interactions environments (Table 6, Appendix 2) with a high nutrient complexity in the military shooting range, Kachia (Figures 9&10), containing multiple resources or niches, can reduce selection for competition particularly if each species is limited by a different resource (Resource Ratio Theory) must rely on evolving or engineering metabolic codependence in a mixed culture (bacteria) for survival and adaptation for bioremediation to occur (Figure 13 & Table 5). Microbiome studies indicate that, in agreement with the ‘habitat filtering’ principle, species with similar resource requirements tend to live in similar areas of the body which explain local competition with the microbial bacteria consortium, Figure 14, Appendix 3. Apart from ecological conditions, different strains can remain mixed in space if they depend on each other for growth and survival based on the as narrated in Table 2, Figure 9. Competition can become neutralised through a reduction in interaction strength, potentially leading to symbiotic relationships and productive communities [115-119]. The dynamics of stability and diversity, then, strongly depend on environmental conditions, and the nature of the competitive phenotypes for collaboration would lead to more accurate and informed predictions on the nature of interactions in microbial communities. The ability to make such predictions can have many important implications in the management and design of microbial communities for xenobiotic biodegradation and understanding of microbial competition for microbial bioengineering narrated (microbial competition and sustained biodegradability, Appendix 3, Figure 14).
To read more about this article...Open access Journal of Biology & Life Sciences
Please follow the URL to access more information about this article
To know more about our Journals...Iris Publishers
No comments:
Post a Comment