Authored by Ruhul A Khan*,
Abstract
Nowadays food irradiation process is an engrained technology for the preservation of foods and food products. It is the process of exposing food or food product to ionizing radiation such as ℽ-rays which is emitted from the radio-isotopes Cobalt-60 and Caesium-137, or electron beams and X-rays. In the food irradiation process, ionizing radiation is use to destroy the harmful biological micro-organisms in food which is recognized as a safe and well-proven process found with many beneficial applications. Based on the absorbed dose of radiation, various effects on foods and food products can be achieved: such as extended shelf-life, ameliorate the microbiological and parasitological safety of foods, reduced storage losses, etc. The most common irradiated commercial products are fruits, spices, and vegetable seasonings. Typically, the food irradiation process is applicable for fresh, dried, and frozen foods with the desire of antimicrobial treatments for spices, fruits, and dried vegetables, insect disinfestations, and control of pathogenic bacteria is in fish, meat, eggs, seafood, and fend off sprouting of potatoes and onions. Nevertheless, the usefulness of food irradiation technology still disputable because of the negative public and industry sensationalism of nuclear technology and the pre-eminence of irradiated foods. This review article is focuses on a brief review of the food irradiation process based on ionizing radiation for the safety and preservation of food and food products. Here, the mechanism of radiolysis of water by irradiation is also discussed.
Keywords: Food irradiation; Ionizing radiation; Food processing; Food safety; Nutrition
Introduction
Introduction In recent years, the food irradiation process has gained tremendous attention among the retailer and food industries to preserve food and food products and assure the safety and nutritional facts as well. This process works for augmentation of shelf-life and microbial safety, preservation of food by disrupting the biotic progression that lead to the collapse of the quality of food and food products. Also, it is very effective in vegetables such as onions, potatoes in reducing the incubation of tubers after harvesting and is competent in delaying the ripening of fruit. Earlier irradiation pro cesses was used for spices and other food ingredients, but for meat and meat products it is now developing into a significant beneficial reality day by day [1-4].
Using ionizing radiation for the food irradiation process becomes one of the efficacious techniques to preserve food with the lowest disruption to the nutritional properties of food products. The irradiation process of food involves the precise application of energy from ionizing radiations such as gamma-rays, X-rays, and electron beams (E-beams). The radiation works to eliminate the water, moistness, and other destructive biological particles in a food system, which mostly works as an oxidizing agent and makes frequent changes in the organic matter of the foodstuff. Similar to other preservation techniques, the irradiation process also causes some physicochemical changes in food and food products. The physicochemical changes are depending on the type of food and food products to which the irradiation process is subjected to and another important one is the dose level of applied ionizing radiation. This preservation process involves exposing the food, either it is pre-packaged or wholesale, to a fixed level of ionization radiation [5-6].
Furthermore, the application area of ionizing radiation in the irradiation process has been extended to enrich the functional properties of food proteins as well. The irradiation process already proved as effective and harmless in disputing immunological and sensitized properties of lectins, the most common agents in food prejudice; hence, it also expected as an effectual procedure to condense the allergenicity of food. Therefore, food irradiation can be considered an evolving technique that is capable of increasing the shelf-life, deferring the ripening of fruits, and thwart the foodborne ailments owing to the declining of non-spore-forming infectious microbes. The study from previous researchers demonstrates that the irradiation process is approved in almost 50 countries around the world for a large number of food and food products [7-9]. In this paper, the application of ionizing radiation in the food preservation process has been discussed.
Food Irradiation Process
Since the beginning of human civilization, there are several procedures have been used to preserve foods and food products. Through the advancement of technology, the procedures of food preservation have also undergone major changes. Technologies for the preservation of food and food products consist of a variety of processes: such as drying, fermenting, pickling and salting and pasteurization or sterilization process, etc. [10]. Among all the food preservation processes, currently, ionizing radiation gained vast attention among consumers and food industries all over the world. The irradiation technology is applied to the food and food products for several purposes such as improving safety, reducing pests that are harmful to food and food product. Table 1 represents the different preservation process of food products.
Table 1: Different Technologies for Food Processing.
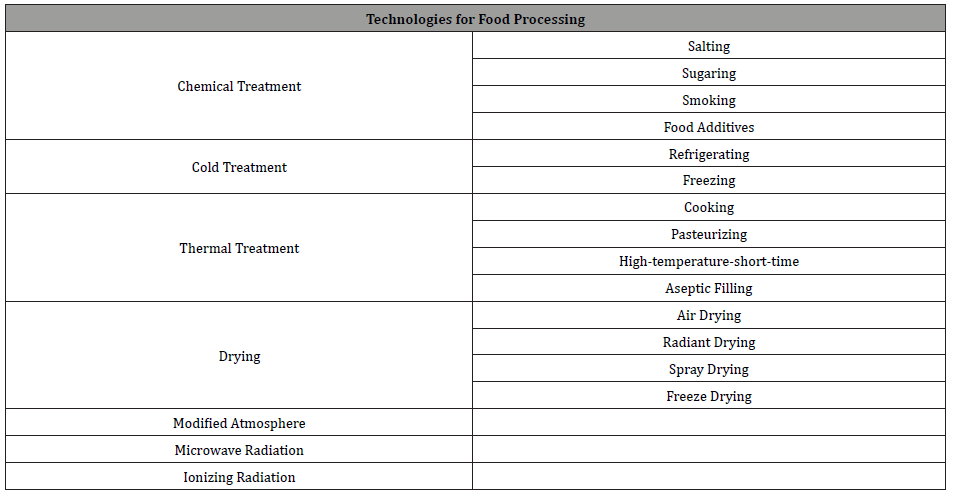
Ionizing radiation for food irradiation process
The most recent method for the food preservation process is ionizing radiation. Over the last few years, ionizing radiation such as ℽ-radiation, E-beams, and X-rays have emerged as the privileged methods for the food irradiation process. The process of food irradiation involves exposing the food and food products to ionizing radiation at a specific dose level. The standard of the International Commission of Radiation Units, the quantity of absorbed radiation dose for the irradiation process is measured in Gray (Gy). Where one Gray is equal to 1 Joule of absorbed energy per kilogram [10- 11]. According to the IAEA reports, there are three types of ionizing radiation are used in the commercial irradiation process to sterilize the foods, medical and pharmaceutical products. These are X-rays, high-energy electron beams (β particles), and γ-rays. These types of ionizing radiations are covered by regarding foods and food elements preserved with ionizing radiation [12].
1. Gamma-rays: Contains energy levels of 1.17 MeV and 1.33 MeV which are emitted by cobalt-60 or Gamma rays; 0.66 MeV is emitted by Caesium-137.
2. E-beams (β particles): Electron beams are usually generated from machine sources. Maximum energy level is not to exceed 10 MeV.
3. X-rays: X-rays can be emitted in accelerators. For X-rays, the maximum energy level is not to exceed 5 MeV.
Each type of ionizing radiation consists of different properties, advantages, and technological drawbacks. Leemhorst and Miller stated that the gamma-rays and X-rays are basically from part of the electromagnetic spectrum, such as radio waves, microwaves, ultraviolet, and visible light rays, etc. Both of the ionizing radiation are consisting of high energy region of the spectrum, short wavelength, and can penetrate foods to several decade centimeters depth [13].
The gamma-rays are generated by using two types of radionuclides such a Cobalt-60 and Caesium-137. Among the two radionuclides, the Cobalt-60 source is most preferable for the irradiation process worldwide. Because of the high solubility of the isotopes in water, the use of Caesium-137 for food processing purposes is highly discouraged. In the case of E-beams, it also has a similar result on atoms and molecules, such as impairment of double-stranded structures like microbial DNA and the formation of exceedingly reactive free radicals. X-ray’s existence is penetrative, which is expansively used to irradiate food and food products that have been packaged. Gamma irradiation is the most preferred method of food irradiation due to the continual emitting of the rays at a predictable rate. The Electron beam and X-rays both of the ionizing radiations are generated by the machine and they are reliant on the parts which can attire out whereas also being liable for a relentless flow of supply of electricity [14-15].
However, during the irradiation process, each type of ionizing radiation primarily interacts with food nutrients and produce similar reactive chemical arbitrates that are fleeting and dissolve rapidly after exposure to the ionizing radiation. The main effects of irradiation are due to the indirect action of these fleeting chemicals instead of the direct effect of radiation itself. A specified dose of gamma-rays, E-beam or X-ray gives rise to a similar effect. Though the ionizing radiation has a destructive effect on micro-organisms in food and food products if it is applied at a specific dose level. The absorbed energy from ionizing radiation deactivates the micro-organisms by damaging the critical component in the cell, usually the chromosomal DNA. The damage prevents growth and arbitrarily terminates most of the cell functions. The effects of damage to the DNA results from direct influence between radiation energy and the genetic material or the interaction between an adjacent molecule and the radiation energy and then it reacts with the DNA [16-17].
The efficiency of a radiation dose depends on the food conformation and the external factors such as the presence or absence of oxygen, moisture content, density, and temperature. Regardless of the absorbed dose, irradiation technology is a low energy process where the product temperature increases by a few degrees centigrade at a high-dose range. Currently, the radionuclide facilities are also used for the treatment of food and non-food applications wide-reaching, such as sterilization of medical supplies and pharmaceuticals, and veterinary products, etc. [15-18].
The electron beam accelerators are also used in the production of some packaging materials and for the plastic wire insulation treatment to expand its properties. As stated by the previous researchers, the food irradiation facilities can be operated in batch and continuous mode. The Batch facilities are most preferable because of the flexibility and ability to accommodate a wide range of doses. When it needs to apply a specific dose range and accommodate the large volumes of a single food product, here the continuous irradiation facilities are better. There is also another facility, known as mobile irradiations. This facility has been used for research purposes for the treatment of seasonal foods such as fruits and vegetables, and fish irradiation onboard ships. Nowadays, the food irradiation process has developed in many countries. Although the most preferred facility is a gamma radiation facility, the use of electron beams is also gradually increasing. There is also interest in using X-rays in the treatment of food and food products. It is to be expected that the majority of the commercial food irradiator facilities will continue to be γ-irradiation facilities for a significant time because of their beneficial appearances [19-23].
Gamma Radiation Facilities
Ionizing radiation is sufficiently high in energy to eliminate the electrons from water, which is the main constituent of foods and living organisms, and to generate highly reactive species with free radicals such as the hydroxyl radical, and hydrogen peroxide. However, the immense mainstream of food irradiation facilities is the γ-irradiation facilities. The γ-rays are basically from radioactive isotopes of Cobalt-60 or Caesium-137 and the most commonly used for food irradiation in a controlled environment [24]. Most of the gamma irradiation facilities are using Cobalt-60 as a radioactive metal that can decay by a half-life of 5.23 years, which means in every 5.23 years the amount of Cobalt-60 will be half in value. Some of the commercial gamma irradiation facilities are used Caesium-137 as a gamma ray source. Although Caesium-137 has a longer halflife of 30.1 years, Caesium-137 emits γ-rays that are nearly half the energy of those emitted by Cobalt-60, and under normal conditions and the Caesium-137 exists in a chemically stable ionic salt as an anion. The metallic form of Cobalt-60 and its higher gamma rays energies offer significant technological advantages for the large irradiation facilities [25-26].
Generally, Cobalt-60 gamma irradiator plants use a radioactive source and generating gamma radiation with 1.17 MeV and 1.33 MeV of energies. The major characteristic of gamma radiation is high penetrating power, which facilitates its use in the treatment of bulk items such as chickens and drums of food. Previously stated that the gamma-rays are emitted impulsively during the radioactive decay of Cobalt-60 and Caesium-137. It is observed as the simplest form of radiation, and photons that are emitted by the radioactive isotopes of cobalt-60 or Caesium-137. Even though the gamma-rays can be simple in concept, but further in practice, it might be challenging. The radioactive isotopes are formed by exposing them to a nuclear reactor core, and the implement is convoluted even after the source is selected, so the source cannot be switched off logistically. Moreover, they do not be an adjunct to the directional or intensity controls. Part of the normal operational technique for a gamma food irradiation facility is to intensification the extent of time that the food is exposed to the ionizing radiation, as the gamma- ray source comes to be older. It is necessary to compensate for radioactive decay and with Cobalt-60 this can be accomplished by a monthly alteration of a few percent to deliberate the speed that the food can travels around the radiation source. The radioactive source has to be refilled from time to time to enhance the source and sustain a suitable processing throughout. The γ-radiation cannot be switched off in emergency cases if it is necessary and the facility is operated around the clock to make full use of the radioactive material [20, 27].
Electron beam facilities: Electron beams or E-beams are mostly produced by accelerating a stream of electrons and produced by the machines, not from the radioactive materials. The high-energy E-beams are produced by an electron gun, and it is easier to direct the electrons with a magnetic field. The E-beams are focused on a thin beam-spot and this spot of incident electrons is scanned athwart the food and food products as it travels perpendicular to the beam direction, through the irradiator. The E-beams delivers dose at a higher rate, and in less than a second can convey a dose that would take hours a gamma irradiation facility to deliver. In divergence to γ-ray facilities, if necessary, the electron beam irradiator can be switched off. However, the electron beams do not infiltrate into the food and food products as like as great a depth as the γ-rays can be penetrated. Therefore, the electron beams are not suitable for treating large bulk packages of food and food products. In this case, the word ‘irradiation’ could be ambiguous as food is not exposed to the electromagnetic radiation or beta rays, but the procedure has a similar effect to gamma ray’s irradiation. During the procedure, shielding is still required but not to the extent of gamma ray’s where concrete bunkers are used [20,22,28].
X-Ray facilities: X-rays are also produced by the machines and they can be switched off if it is necessary, as with E-beams. Here electrons are accelerated at a metallic target and this generates a stream of X-rays. The process is not effective, but the X-ray renovation efficiency surges with a cumulative atomic number of the metallic targeted substantial and with increasing the incident E-beam energy, whereas most of the E-beam energy are lost as heat. According to previous research reports, currently, very few foods are irradiated by X-rays. Nevertheless, X-ray irradiation is finding more favors associated with E-beams and other radiation processing applications as they are more pungent than the E-beams, gathering it probable to process the large wholesale packages but without the stipulation for radioactive material. X-ray irradiation will likely become more widespread in the future as technology advances. Relatively a new technique, includes exposing the food to higher energy photons which possibly have a deeper penetration depth than the γ-rays. The big advantage of X-ray radiation is it can be switched on and off, yet when it is on, the shielding is necessary but again not to the extent of gamma rays. This process does not result in any radioactive substances or by-products [23,29,30].
Application of radiation for food processing
The ionizing radiation is commonly used for food preservation purposes. Based on the previous research reports, the major benefits are achieved by using ionizing radiation for processing of food including sprouting inhibition, delayed ripening and senescence of fruits and vegetables; disinfestation of insect pests in agricultural commodities; destruction of microbes responsible for spoilage; elimination of pathogens, and parasites of public health concern. Radiation augments the keeping quality of certain foods through a considerable reduction in the number of spoilages causing microorganisms [31,32]. The irradiation process depends on the radiation dose levels, such as the low radiation dose range of 0.02-0.2 kGy, the sprouting of potatoes, onions, garlic, shallots, yams, etc. can be inhibited. The 0.2-1 kGy dose range is used for delayed physiological processes such as the ripening of fruits. Depending on the product, in such treatments 1-7 kGy dose range is used to exposed fresh meat and seafood [32,33].
In the food irradiation process, the applied dose level can be divided according to the type of food and food products. For example, for fresh fruits and vegetables, a low dose of up to 1 kGy is used to delay ripening and sprouting in addition to controlling pests and foodborne pathogens for dried fish and meat, fresh and dried fruits, etc. Medium dose level, from 1 to 10 kGy is used to reduce the spoilage and pathogenic microorganisms of fresh fish, strawberries, mushrooms, seafood, poultry, and meat to expand technological properties such as reducing cooking times for shrivelled vegetables and extending the shelf-life of the foods and food products. The high dose level of radiation is applied for the sterilization purposes of meat, poultry, seafood, and other ready foods in amalgamation with insignificant heating to inactivate enzymes and for microbial disinfection of some specific foods and ingredients such as spices, seasonings, and enzyme preparations, the applied dose level for sterilization is greater than 10 kGy (10-50 kGy). The most important benefit of food irradiation facilities is it does not become radioactive and does not create radioactive wastes. According to previous research findings, currently, there has been growing appreciation of the importance of irradiation technology in the food industry in both developed and developing countries. It has been reported that there are more than 50 countries have already given sanction for over 60 food and foodstuffs to be irradiated for local consumption and for export purposes, and approximately 40 different countries are now using the food irradiation technology. The food irradiation technology is already privileged in the USA, South Africa, Netherlands, Thailand, and France [7,24,34]. Table 2 and Figure 1-4 shows the benefits and applications of ionizing radiation in food processing based on different dose levels [31,32].
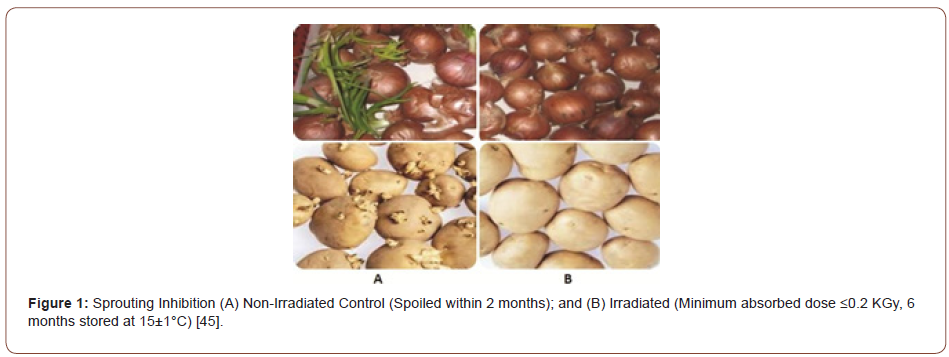


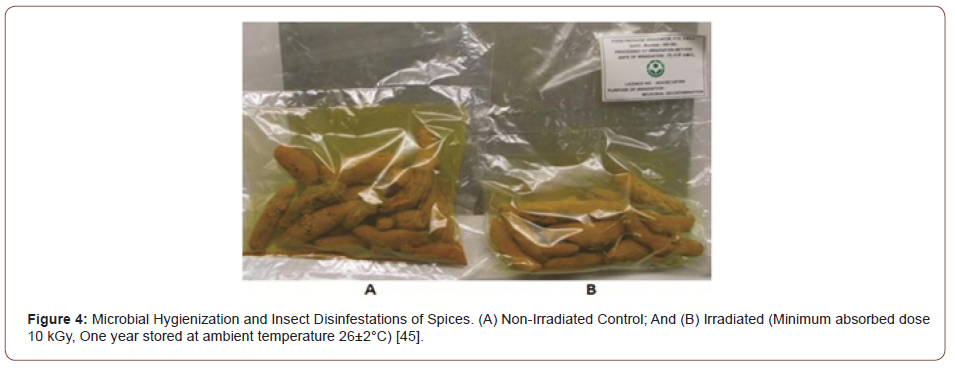
Table 2: Radiation Dose Applied in Food Industries, Associated Benefits and Products [11].
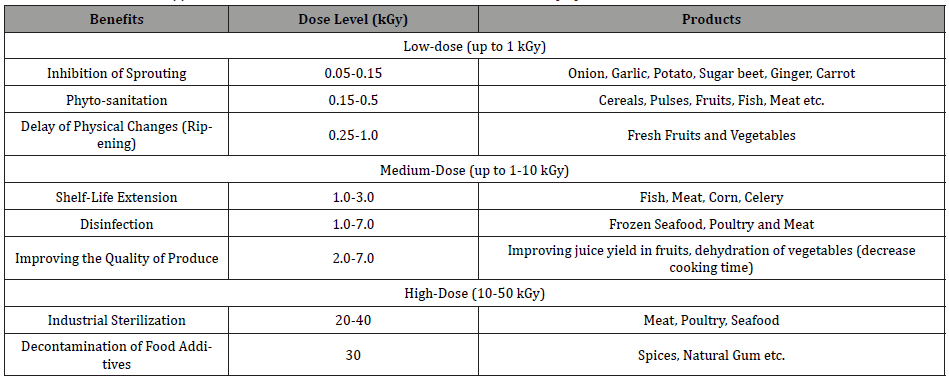
Sprouting inhabitation: Based on the research of Ahari and Safaie, to supply the root vegetables and spices such as potatoes, garlic, onions, and yams, it is necessary for consumers to go through the irradiation process to inhibition of sprouting for a year-round supply and storage duration up to several months [35-37].
The refrigeration process also can be applied for sprouting inhibition and the application of various chemicals such as hydrazide and isopropyl chlorocarbamate for preharvest and postharvest foods. The chemical treatments are comparatively inexpensive and effective than the refrigeration process. Due to health issues, many countries have banned their usage. In such instances, the irradiation process can be indorsed as a reasonable substitute for the chemical and refrigeration processes. At the high dose range of 0.5-1.5 kGy; sprouting inhibition, reduced decomposing and weight loss have been observed in potatoes, garlic, onions, and yams, etc. [38-40].
Insect disinfestations: Before 1885, the fruits and vegetables from pest-ridden areas were disinfected by chemicals and the best controls for insects in grain products can be achieved by using ethylene dibromide or ethylene oxide [40,41]. However, due to the concern of health and environmental issues, the usage of these chemicals has been barred or strictly restricted in most of the countries around the world. Whereas the heat and cold treatment procedures can be used as a proficient of insect disinfestations, they can also penetratingly reduce the taste and appearance of the food and food products [39,42]. Therefore, recently the irradiation process has been endorsed as a substitute for disinfection. Disinfestations are intended at averting the losses that are caused by the pests in stored grains and grain foodstuffs, pulses, flour, cereals, coffee beans, dried fruits, nuts, and dried fish, etc. [41,43]. Previous researches stated the practical experience shows that the required radiation doses are in the range of 1.5-7 kGy. At 0.25kGy dose level can be effective for quarantine treatment of fruits, while 0.5 kGy dose level can control all stages of pests [43-45].
Foodborne pathogens: The procedure of enlightening the sterilized quality of food and food products by defusing the foodborne pathogen microbes and pests is called radicidation. This process of oscillating the shelf life is called radurization and utilizes the medium dose of radiation, usually 2-8 kGy; it is similar to the heat sterilization process, and therefore it is called radio sterilization. For the microbial decontamination of dried spices and other dried vegetable seasonings, the irradiation process at high dose levels (10-30 kGy) of ionizing radiation is an effective substitute for chemical fumigants like ethylene oxide. This is achieved by reducing the total microbial loads such as pathogenic micro-organisms of the food and food products. The sterilization process of ionizing radiation is achieved by lessening the activity of all the micro-organisms of food spoilage in the treated product. This process is analogous to the thermal canning in attaining the shelf-stability and it is called radappertization [31,46,47].
For foodborne illness, beef, pork, poultry, seafood, eggs, and dairy products all are recognized as the major sources. For beef, the most serious contaminants are Escherichia Coli or E. coli, listeria, and tapeworm and for poultry meat and eggs, the most important pathogens are salmonella and campylobacter. For all these organisms, an excellent control can be achieved with the radiation dose range of 1-3 kGy [48-50].
Spice irradiation: The spices which are derived from the fresh plants are almost contaminated by the microorganisms; like from soil, with blown dust, and by the bird droppings. During the drying process of spices, these microorganisms can endorse to population densities phenomenal 106 organisms per gram of materials [39]. When the spices are used as seasonings in the manufacturing of the processed foods, these micro-organisms can cause rapid food spoilage and can lead to foodborne illness. Since for the moist, generally, the heat treatment process is not suitable for such dry products, in the past spice producers routinely used fumigants for disinfestations. For this reason, the producers are now increasingly turning to ionizing radiation treatment. In fact, the commercial irradiation treatment of spices has been permitted and has already been in operation in many countries for several years. The medium dose level of ionizing radiation (5-10 kGy) usually gives quite satisfactory results for the elimination of bacteria, mold spores, and insects without any kind of negative impacts on chemical or sensory properties [43,51].
When the products are exposed to high radiation dose levels; usually more than 10 kGy, some foods such as fresh fruits and vegetables have deteriorated. However, the other foods including seafood, meat, and poultry do maintain their good quality, provided that certain safety measures are taken. As a result, it is possible to effectively sterilize these foods with high doses in the range of 25- 45 kGy [42,52,53]. To prevent off the flavors resulting from lipid oxidation, the oxygen must be excluded by the vacuum packaging and the irradiation process must be performed at low temperatures (-20°C to -40°C). Foods that are preheated to inactivate the enzymes can be commercially sterilized such as it occurs in canning. These products can be stored at room temperature almost indefinitely. While these additional procedures and high dose levels eloquently increase the costs compared to the other processes, these products are even so important for the hospitalized patients with suppressed the immune system and the NASA astronauts [48,54].
Effects of Ionizing Radiation on Food
The irradiation process produces a little or no chemical change in the food and food products; the physical properties of the food and food products are also not affected by the irradiation. The majority of changes of the food and food products due to the irradiation processing are similar to those by the other preservation methods such as thermal food processing. In general, the extent of the chemical reactions induced by the irradiation in food components depends on many variables. The most important variables are the irradiation treatment conditions, such as the absorbed dose level, applied dose rate, facility type, presence or absence of the oxygen, and temperatures. The composition of the food and food products and their physical states such as the frozen or fresh, solid, liquid, or powder have also impacted the extent of the reactions tempted by the radiation treatment and the nature of the formed products. The effects of irradiation can be affecting the nutritional quality and shelf life of the food products [45,55]. Some of the most important changes carried about by the food irradiation process in nutritional quality, shelf-life extension, and the toxicology of the irradiated foods are further discussed in the following sections.
Shelf-life extension
The shelf-life extension of the food products is attained by inactivating the micro-organisms present in them. The radiation, irrespective of its nature such as ionizing or non-ionizing, causes damage to several components of the cell, including the genetic materials of the food and food products. This not only disrupts the cellular functions of the food products arbitrarily but also incapacitates the ability of micro-organisms to imitate or regenerate. Furthermore, the ionizing radiations are also subjected to the interactions of other components of the cell of the food products. Even with an undisturbed DNA, the damaging effects on the cellular proteins and the membrane may hamper the chances of survival of the injured cell. However, some certain bacterial types have altered to resist the changes carried about by irradiation [56]. For example, Deinococcus radiodurans is a bacterial species that can resist at high radiation dose levels up to 30 kGy. Due to its highly efficient DNA repair mechanisms, this bacterial species can survive moderately at high doses of radiation. In DNA repair mechanism, the deinococcus radiodurans can converse the effects of radiation damage on its DNA utilizing an effectual proteome mechanism that empowers the DNA repair; including the disintegrated DNA [57]. Due to the flaws in chemical treatments, the appearance of the multidrug- resistant microorganisms is a concern and this spectacle has drawn counterparts with the irradiation process. Nevertheless, the micro-organisms of irradiated food and food products are sensitive for treatments involving the temperature variations, the antimicrobial components of foods, and the repeated irradiation process. Over recent years, there are several countries across the world adopting the irradiation process to treat and preserve the food and food products of various natures, studies of this technology for the shelf-life extension have enhanced [30,58].
For the foodborne pathogens, the same radiation dose levels are appropriate to extend the shelf-life of the food products significantly by reducing the populations of spoilage bacteria, molds, and yeasts. For example, Patterson reported in 2005 that to extend the shelf life of chicken and pork by as much as a few weeks, the applied radiation dose level is 2.5 kGy; on the other hand, if 5 kGy dose level applied to the low-fat fish the shelf-life can be extended from 3-4 days without irradiation to several weeks. Besides, the shelf-life can be also extended significantly of various cheeses by eliminating molds at less than 0.5 kGy dose level. Finally, the extension of shelf-life for strawberries, carrots, mushrooms, papayas, and packaged green vegetables also appears to be promising at a few kGy dose levels or less [36,59]. The irradiation process of mushrooms at the radiation dose level of 2-3 kGy inhibits the cap opening, the stem elongation and can be increased at least two-fold by storage at 10°C. The treatment of strawberries with a dose level of 2-3 kGy, followed by storage at 10°C can result in a shelf-life extension of up to 14 days [35]. Ripening fruits such as bananas, mangoes, and papayas can be delayed by the irradiation at a dose level of 0.25-1kGy. It is important to irradiate the fruits before they are ripening start [38,39,59].
Nutritional aspects
The nutritional aspects of the food and food products are the most important things to be considered during the preservation process. Irradiation is a cold process that does not involve any temperature rise. The food irradiation process has no effects on the nutritious values of the food products, and it does not affect its significant macronutrients; such as, proteins, carbohydrates, and fats, hence leaving the food fresh. The changes conveyed to the nutritional carbohydrates, such as starch, have been expansively studied over years. In recent studies, the deviations in functional properties of starch and wheat flour after ℽ-irradiation has been investigated. It has been reported that there were no substantial variations in the composition and bulk density of the flour. Depending on the dosages, the amylose contents in the flour increased by 25 to 36% [11, 60]. An interesting study was conducted on the effects of the ℽ-radiation and E-beams to conserve the wild Arenaria Montana L, which is an important source of bioactive compounds. Furthermore, a decrease in the viscosity was also observed, convoyed by an increase in water and oil absorption capacity. Depending on the composition, irradiation leads to oxidation, polymerization, decarboxylation, and dehydration in fatty acids, lipids and releases several compounds. Both treatments had a substantial effect on the fatty acid content [61].
While the drenched fatty acid and monounsaturated content are amplified, a reduction in polyunsaturated fatty acids was observed. Many scientists have reported in several types of research that the effects of ℽ-radiation and E-beam radiation on the chemical and antioxidant profiles of numerous food products. The most noteworthy variations in sugar, protein, fatty acid and antioxidant activity in Amanita mushrooms were recorded when it was exposed to the E-beam radiations. Due to the increase in nitrogen atoms that are caused by the excision of C-N bonds or protein unfolding which takes place during the Kjeldahl reaction, translating into higher nutritional content the apparent increase was in the protein contents of the irradiated mushroom. Treating mushrooms using 2 kGy gamma radiation can lead to a reduction in sugar content [33,34]. The ℽ-irradiation and E-beam irradiation decrease the antioxidant activity in the wild Arenaria Montana L [61,62]. The Arenaria Montana L contains apigenin derivates which are bioactive that can exhibit anti-inflammatory and anti-cancer properties [63]. For vitamin A and E, milk and dairy products are considered as the major sources, but these food products are usually not suitable for irradiation treatment. However, irradiation treatment of dairy products can be carried out up to 40 kGy without forming any off flavors provided that the temperatures for the procedure are maintained as low as -78°C [64]. The precursors of vitamin A, Carotene, and beta- carotene are mostly not affected by the irradiation of fruits such as Lycium [65]. However, this result can vary according to the type of fruits and vegetables. For example, 50% loss in beta-carotene content happened when carrots were irradiated by using 0.1 kGy doses and stored for 6 months [66].
Toxicological aspects
The toxicological aspects of foods due to exposure to ionizing radiation are considered another important fact of the irradiation process. While the reactive radicals generated by the food irradiation process results in improved the shelf-life, it can also react with the chemical mechanisms, producing radiolytic products, such as short-chain hydrocarbons and formaldehyde. Radiolysis of triglycerides increases to 2- alkyl-cyclobutanones (2 ACBs). Since these molecules are exceptional, they have been preferred as indications to identify the food products that have been subjected to go through the irradiation process. The exceptionality of these compounds has triggered studies for their identification [67-68].
Over the past decade, different studies have been conducted to determine the toxicology and mutagenic effects allied with the consumption of 2-ACBs. It has been reported that at the low absorptions, 2-ACBs exhibit no mutagenic or genotoxic effects on mammalian cell lines. Yet, the consumption of these chemicals at higher doses has resulted in cytotoxicity and damage to the genetic material in rat and human colon cells. The irradiation process can also control the development of nitrosamine and nitrite-related products in cured meat. Nitrates and nitrites are the food additives in processed meat that impart color and flavor. It has been revealed that these additives are also potential cancer-causing agents. Irradiating cured meat at prescribed sterilization doses eradicates or declines the levels of nitrates and nitrites considerably to maintain the color and flavor of the meat product. Also, there are several studies have been conducted in vitro on the possibility of chromosomal damage that may occur due to the consumption of irradiated foods [11,69,70].
Safety aspects
Food Irradiation technology has been officially accepted by international organizations due to the effectiveness in food, wholesomeness, and economic benefits. Overall, many international organizations such as the World Health Organization (WHO), the Food and Agriculture Organization (FAO), and the International Atomic Energy Agency (IAEA) have a less preventive tactic than the European Union. In 1981, it has been reported that 10 kGy dose level was set as the maximum dose considered to be safe and wholesome by the join expert of FAO, IAEA, and WHO committee on the wholesomeness of irradiated food and food products. The energy transported up to 10 kGy did not show the toxicological risk without significant damaging effects from a nutritional or microbiological viewpoint. Another opinion from the same committee that it is suitable to specify a maximum dose level for the treatment by the ionizing radiation of certain food products and that irradiated foodstuffs should continue to be estimated separately taking into account the scientific need and their safety. The safety of the food and food products or raw matters employed as ingredients submitted to the ionizing radiation nonetheless of the purposes for which the treatment is carried out is validated by the scientific evidence [71]. However, in vitro studies have detected the tumor-promoting activity of 2-ACBs. Due to the lack of sufficient data on the effect of long-term consumption of irradiated foods on human health and on long-term health effects of eating a diet based on the irradiated foods, it is still considered to be a problem; preventive principles should be applied until such data are available. Recently, a scientific opinion of the European Food Safety Authority (EFSA) regarding the chemical safety of irradiation treatments of food concluded that most of the radiolytic products as certain hydrocarbons, 2-ACBs, cholesterol oxides, and furans are also formed in food products that have been subjected to other food processing treatments and are thus not exclusively formed by irradiation. Furthermore, the amounts in which they ascend in irradiated food were not significantly higher than those being formed by the heat treatments. The bulk of indication from the recent literature concerning some biological effects supports the food classes and radiation doses specified, based on previous opinions that are provided by the Scientific Committee on Food of European Commission [7,10,72].
Induced Changes on Food Components
The irradiation process causes variation both in the DNA of living cells and food molecules. The main effects are the development of ions and the free radicals, breaking of chemical bonds of the food molecules, and neoformation of the constant molecules. The damage and modifications at the DNA level of micro-organisms can extinguish the cell, which is the main effect of radiation on food such as the destruction of insects and the inactivation of pests. The secondary changes in irradiated foods are those associated with the modification of flavor. This effect is the significance of lipid oxidation, the appearance of mercaptans and diminution of free radical scavengers like vitamins C and E. Hydrocarbons and 2-alkylcyclobutanones (2-ACBs) are the main radiolytic products that are produced from the major fatty acids in foods, and some cholesterol oxides and furans [73,74]. Moreover, the application of ionizing radiation in the food preservation process has been extended to the enhancement of the functional property of the food proteins [75]. The irradiation technology was proved as effective and safe in combating immunological and allergic effects of lectins, the most common agents in food intolerance; therefore, it has been proposed as a method to reduce or eliminate food allergenicity [71,76]. The potential for application of irradiation as a food preservation processing technology to diminish the undesirable and toxic compounds in the food and food products, such as N-nitrosamine, biogenic amines, and residual nitrite, has been reviewed in many researches. The demonstrated efficacy of ionizing radiations in reducing biogenic amines in ripened cheese during 6 months of storage has been reviewed recently [77-79].
The Radiolysis of Water
Because foodstuffs generally contain a significant amount of water, a short discussion of the radiolysis of water by irradiation with ionizing radiation (primarily γ-photons but also X-rays, UV photons, electrons, and neutrons) is necessary. Non-particle ionizing radiation (γ-photons and X-rays) is electromagnetic radiation of very short wavelength (high frequency) as depicted in Figure 5.
A huge literature exists on this subject so that only a few general sources are cited. The subject has been extensively explored because the radiolysis of water generates highly reactive species, including eaq -, H, OH, H2O2, HO2, HO2 -,O2, O2 -, O2 2-,O-, O2 +, O, H2, OH-, H+, and possibly others [81]. These species are either oxidizing agents (e.g., O2, O2 + ,H2O2, OH, O, O-) or reducing agents (H2, H, eaq -, O2 2-, O2 -, O2 2-) some of them being thermodynamically quite powerful, as measured by the standard reduction potentials summarized in Table 3. The table also contains nitrogenous species that may be generated via the homogeneous decomposition of N2 into highly reactive nitrogen atoms which may then react with water and water radiolysis products to generate a range of other species, including NH3 and the oxyanions. In neutron irradiated systems, nitrogen is also produced by the nuclear reaction 16O8(1n0,0e-1)16N7 with 16N7 being radioactive. Because of neutron activation phenomena and the general unavailability of neutron sources except for research purposes, neutron irradiation is of little interest in the food industry.
Table 3: Radiation Dose Applied in Food Industries, Associated Benefits and Products [11].
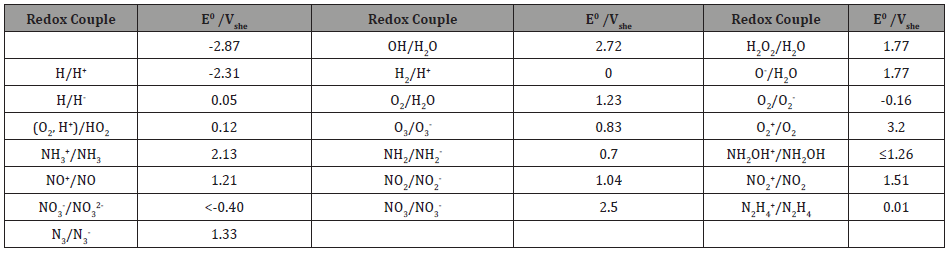

Numerous models have been proposed for the radiolysis of water and one such model that has been employed to model the coolants in water-cooled nuclear power reactors is presented in Table 4. This particular model has been cleansed of unacceptable, non-elementary reactions, such eaq -+ eaq --+2H2 O→H2+2OH-, which may be decomposed into two elementary reactions [2(eaq -+H2O→H+OH-), H+H→H2] that were already included in the original model. Furthermore, such a reaction postulates reaction between species of like charge, which are discounted based on coulombic repulsion. As shown, the simplest way of removing these reactions from established codes is to simply set their rate constants equal to zero.
Table 4: Model for the radiolysis of water as modified from the References [82] and [83]. to model the primary coolant chemistry of BWRs, with the rate constant of Reaction (30) being used as the “calibrating” parameter.
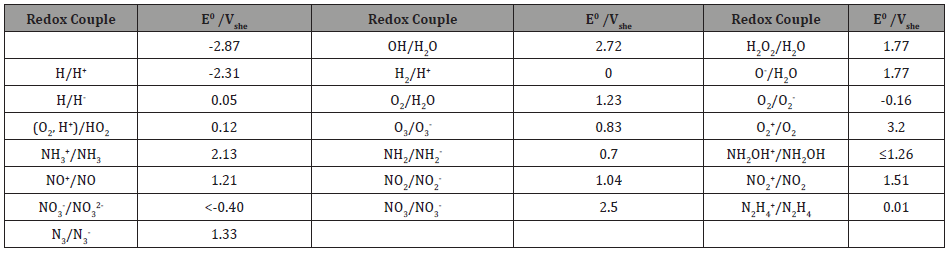
All models comprise a source term of primary radiolysis products that are envisioned to form within the spurs (track of the ionizing particle) in < 10-12 s [80], including eaq -, H2O+ (H+), H, OH, OH-, H2sub>, and H2O2, and a set of reactions between these species at longer times as they diffuse from the spurs to produce the non-primary radiolysis products (Table 4). Accordingly , so that reactions are not counted twice, the radiolytic yiels (G vales, # of a given species that are produced per 100 eV of energy absorbed by the water) must be primary yields. A set of G-values for low linear energy transfer radiation like γ-photon radiation are summarized in Table 5. Note that the yields for secondary species have been set equal to zero in an attempt to correct for the fact that they are not primary products that are generated within the < 10-12 s timescale. However, there is no assurance that the G-values are in fact primary yields and it is known that the yield of eaq - and OH, for example, decreases sharply with time over a few nanosecond range [84-85]. Accordingly, only yield that are determined using sub-nanosecond pulse techniques are arguably “primary” yields and, perhaps, the true primary yields are unmeasurable using currently available techniques. Nevertheless, such models have been successfully used to calculate properties, such as the electrochemical corrosion potential (ECP) of alloys in nuclear reactor coolant circuits, for example.
Table 5: G-values (no./100 eV) for low LET radiation (γ radiation) at different temperatures by using relations from [86].
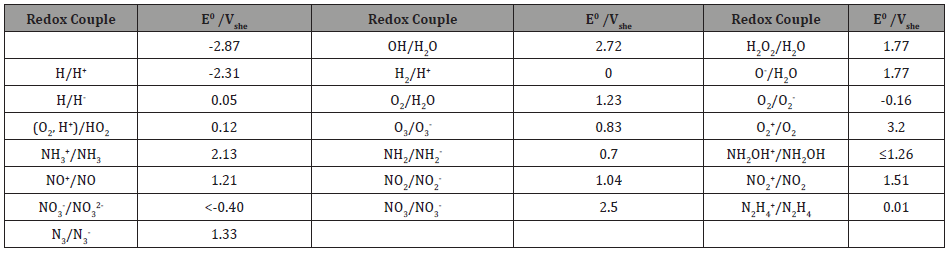
Table 6: Sensitivity of Eredox to [H2*], [O2*], and [H2O2*] for irradiation of water with γ-photons at a dose rate of 1mGy/s at 25°C.
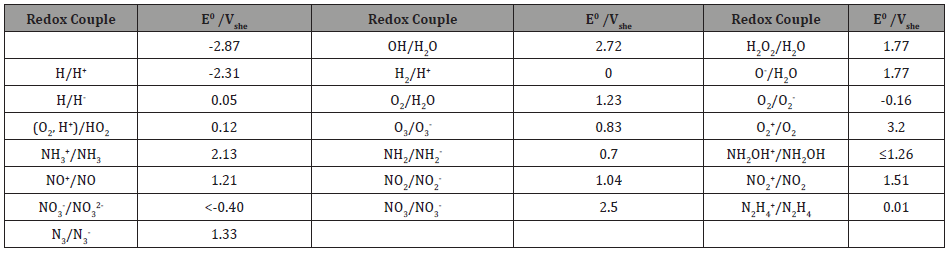
The interaction of ionizing radiation with biological systems has been studied extensively and a comprehensive review has been published [87]. Briefly, the interactions can be classified into direct interactions and indirect interactions. In direct interactions, the IR interacts directly with the biologically active molecule (e.g., DNA, amino acids, etc) primarily via scissoring of C-S-H, C-O-H, and C-N-H bonds to produce free radical and other species, while the indirect interactions result from the radiolysis of intercellular water to produce highly reactive products that then react with the biologically active components of the system, as briefly described above. Specific issues in the IR preservation of food have been reviewed by Catanescu and Tofana [88] while the international standards are articulated in Ref 89. The dose rates employed in γ-photon (60Co) irradiation range up to 0.5 Gy/s, depending upon the food and the microorganisms present, although many dose rates are apparently much lower (e.g., 1.12x10-3 Gy/s [90]). In any event, at a dose rate of 0.1 Gy/s it takes 500,000 s (139 hrs) to accumulate a 50 kGy dose, which is the upper end of the range employed in the food industry.
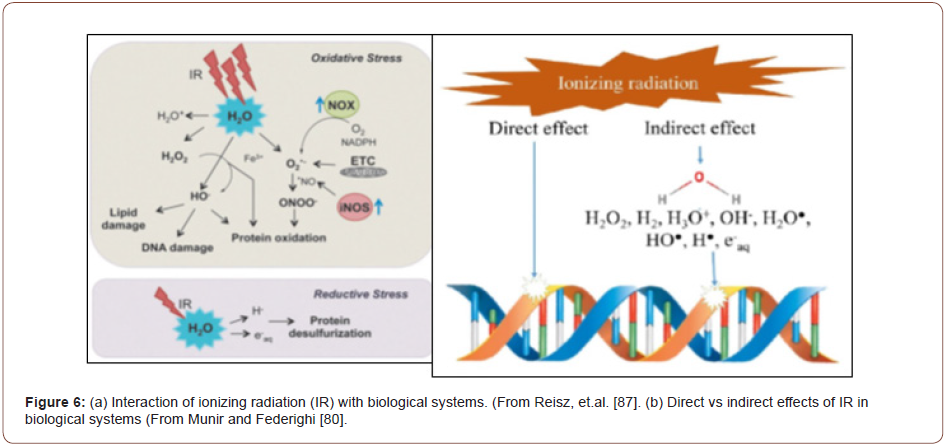
A cartoon depicting the effect of IR in biological systems is presented in Figure 6 [87]. As shown, the indirect effect involves the attack by radiolysis species on lipids, DNA, RNA, and proteins, amongst others entities, primarily by the oxidizing species, such as OH and H2O2O, which are powerful oxidizing agents (Table 2) and O2 - that is a mild reducing agent but which produces a strong oxidizing agent (O2). Of course, the actual processes are much more complex that those depicted in Figure 6, but the general idea is conveyed. Many biochemical processes are redox sensitive [87,88,91]. From an electrochemical viewpoint, the driving force for redox processes is the redox potential (Eredox), which is a mixed potential that is established by a balance of the partial anodic and cathodic processes at an interface such that the net current is zero. Often, this potential is erroneously identified with an equilibrium potential as calculated using the Nernst equation [92-99]. Instead, Eredox can be calculated using a mixed potential model (MPM) [100] that was originally developed for calculating the electrochemical corrosion potential (ECP) of steels in water-cooled nuclear reactor coolant circuits. In that application, Eredox provides a measure of the driving force for corrosion reactions, such as metal electrodissolution and passivation to occur. In fact, to a good approximation Eredox may be thought of as being equivalent to the potential applied between the tip of the Luggin reference electrode probe and the metal in a classical electrochemical potentiostatic experiment. However, it is important to recognize the difference between Eredox and the ECP (corrosion potential) as the former refers to a system for which the anodic current due to the dissolution of the substrate is zero while the latter corresponds to a system in which the substrate is undergoing electrodissolution (corrosion). Since redox potentials are commonly measured with Pt or Au indicator electrodes, this non-reacting substrate constraint is practically satisfied especially because both substrates display catalytic activity toward charge transfer (REDOX) reactions, such as H2/H+ (HER), O2/H2O (OER), and Fe2+/Fe3+, where HER and OER indicate the hydrogen and oxygen electrode reactions, respectively. Accordingly, the exchange current densities for the partial reactions are very much higher than for the same reactions on stainless steels, for example, and these affect the value of the measured potential. An example of the calculated Eredox vs [O2] at 25 °C in pure water containing 1 ppm of hydrogen is shown in Figure 7. Also plotted are the equilibrium potentials for the oxygen electrode reaction (OER) and the hydrogen electrode reaction (HER) for comparison with Eredox. As seen, Eredox displays a sigmoidal variation with composition ([H2]/[O2]) and approaches EHER e and EOER e asymptotically at limitingly low and high [O2], respectively. Note that EHER e is independent of the composition because, in this calculation, the hydrogen content is fixed (1 ppm) while EOER e becomes more positive as the oxygen content of the solution increases, as specified by the Nernst equation. Furthermore, the reader will note the large difference between Eredox and EHER e and EOER e except at the composition extremes where the redox potential is determined by the equilibrium potentials of one of the redox reactions. This accounts for the frequently observed disparity between the measured Eredox and that calculated by using the Nernst equation, which is a common but erroneous practice in biology.


Another issue that complicates our ability to calculate Eredox is the fact that no exchange current density or transfer coefficient data are available for the energetic radiolysis species, such eaq -, H, OH, HO2, HO2 -, O2 -, O2 2-,O-, O2 +, and O. However, a general rule of thumb that has been gleaned by extensive modelling and experimental work in this area is that the contribution that any species makes to the redox potential (or to ECP) is roughly proportional to its concentration and, because the concentrations of these radiolytic species are orders of magnitude lower than those of O2, H2O2O, and H2, their impact is minor (see below). Thus, the redox potential in irradiated systems is dominated by O2, H2O2O, and H2 but that does not imply that the more energetic species are not important in redox reactions since, while their concentrations are low, they are generally of much higher reactivity than are the dominant species. Furthermore, because of their low concentration, the partial currents due to the redox reactions involving these highly active species are mass transfer limited and hence insensitive to the kinetic parameters. The most effective way of addressing this issue is to define new concentrations as [H2*] = [H2] + 0.5[H] + 0.5[eaq -] + [O2 -], [H2O2O*] = [H2O2]O2 + 0.5[OH] + [HO2 -] + [O2 2-] + [HO2] and [O2*] = [O2] + 0.5[O]. One of the authors (DDM) has developed a code, RAD_REDOX, for calculating the redox potential in irradiated systems from similar codes that he developed for estimating ECP in nuclear reactor coolant circuits [98] and these calculations are described later in this review.
Using the methods outlined above, we show in Figure 8 the calculated evolution of the composition of irradiated water at 25 °C for a γ-photon dose rate of 100 mGy/s, which is somewhat higher than the dose rate employed by Britto et al. [90] (1 mGy/s) in their experimental work on the effect of radiation dose rate on psychrotrophic bacteria, thiobarbituric acid reactive substances, and sensory characteristics of mechanically deboned chicken meat. The calculations are for a closed, liquid phase system (no gas phase) and it is seen that the system comes to a steady state after an irradition time of 105 s (27.8 hrs), with this time depending strongly upon the dose rate and hence on the rates of the various reactions in the radiolysis mechanism (Table 4), because the rates of those reactions depend upon those same concentrations. Because the concentrations of the electroactive radiolysis products change with time, so will the redox potential and, hence, the lethality of irradiation toward microorganisms. This issue will be explored in a later paper.
The concentrations of the species then remain invariant from about 10ms up to the maximum simulation time of 104 s (2.8 hrs). Radiolysis, at least at this dose rate, is predicted to have little impact on pH. The dominant species is predicted to be H2, followed closely by H2O2 > O2 > OH ≈ O2- > H > eaq- ≈ HO2 > HO2- in abundance. The redefined concentrations, [H2*] = [H2] + 0.5[H] + 0.5[eaq-] + [O2-] + [O22-] , [H2O2*] = [H2O2] + 0.5[OH] and [O2*] = [O2] + 0.5[O] + [O2+] are calculated to be 2.546x10-7 M (0.0005137 ppm), 1.327x10-7 M (0.004514 ppm), and 5.570x10-8 M (0.001782 ppm), respectively. These concentrations result in a Eredox = 0. 0779 Vshe. Had we used [H2], [O2], and [H2O2] as inputs instead of [H2*], [O2*], and [H2O2*], Eredox = 0.0775, only 0.0004 Vshe lower than if the contributions of the highly energetic radiolysis products (H, eaq-, O2-, and OH) are ignored. This demonstrates the previous conclusion that only the most dominant redox species (H2, O2, and H2O2, in this case, see Figure 8) need be considered in calculating the redox potential (and the ECP) [100]. From the value of the calculated redox potential (0. 0779 Vshe), irradiation of the water with γ-photons at a low dose rate of 1mGy/s at 25 °C renders the system moderately oxidizing, which is attributed primarily to the formation of H2O2.
By calculating Eredox for various combinations of H2*, O2*, and H2O2*, we find that the two dominant species in determining the redox potential are H2* and H2O2* (Table 6). Thus, in the absence of H2 (Low H2* case), Eredox is very high (0.8629 Vshe), which is attributed primarily to H2O2*, demonstrating the powerful impact of the reducing species (H2*) in the system. The impact of H2O2* is further illustrated by the Low H2O2* case where the redox potential is predicted to fall to -0.0085 Vshe. Low O2* results in a modest increase in Eredox from the lor H2O2* case but the dominant roles played by H2* and H2O2* are still evident.
Finally, we can say that the redox potential is an important factor that determine reactivity in irradiated aqueous system, such as food. Other factors controlling the rates of reactions between water radiolysis products and reactive moieties in substrates include the electron density at the reaction site and the activation energy.
Conclusion
Ionizing radiation for the food preservation process has been studied for several decades that it can be used to improve the safety of food and food products. Nowadays, the food irradiation process is a well-known procedure to reduce harmful microorganisms to attenuate foodborne diseases. It is a safe and effective method of processing food and food products. Report of several studies shows that after exposing at specific dose levels the effects of ionizing radiation on approved food and food products eliminated the harmful microorganisms including the food poisoning bacteria such as E. Coli, salmonella, and various types of dangerous foodborne illnesses. The irradiation process delays sprouting and ripening so the food can be preserved for longer without significant loss of vitamin or causing any dearth. The food irradiation process is fully enclosed, and it can be returned for recycling or disposal. This process also has a good safety record and it does not affect the environment or in human bodies as the radioactive materials. Also, the food does not become radioactive as well and there are not sufficient identified cases regarding potential health problems associated with food that has been irradiated. The main challenge of the irradiation process is the consumers and general public attitude towards the implementation of ionizing radiation in the food preservation process. The acceptance of irradiation technology is a matter of proper education, information, and communication to fading the misinformation that food irradiation is using nuclear energy. The general public believes that exposing food and food products to ionizing radiation clutches health hazards and it can be a threat to developing mutant organisms in their body. Some people are also fear about the possible devastating accidents at the food irradiator plants that if more food irradiators are approved then it will increase the risk of accidents. On the other hand, the prices for irradiated foods are slightly higher compared to the other foods. Another problem is the consumer’s acceptance, there are several studies has been conducted based on consumers attitude about the irradiated food products. The research findings have constantly shown that many consumers have misconceptions about the irradiation technology and believe that it makes the food radioactive. Based on the results of different marketing trials, initially, there are 8.4%, after the first statement 28.3% and 42.2% consumers become strong buyer after the second statement. So, results show that if the consumers are aware of the irradiation procedure and its effects on food, then the purchase rate of irradiated food and food products will be increased. The main obstacle to acceptance the irradiation technology is the lack of information about it and its benefits has directed to many controversies. So, it is needed to provide correct information and utilize the food irradiation process as an alternative technology, safe and advantageous process for preservation of food and food products among the consumers, so that they can make informed choices. In conclusion, the misinformation about using irradiation for the scams and humanity should be removed now, and it is high time to utilize this amazing resource of ionizing radiation as irradiation technology for the food preservation process to enhance global food security and safety. Regarding the radiolysis of water by irradiation, it is emphasized that the redox potential is only one (but an important one) of the factors that determine reactivity in irradiated aqueous system, such as food. Supplementary factors controlling the rates of reactions between water radiolysis products and reactive moieties in substrates are the electron density at the reaction site and the activation energy.
To read more about this article...Open access Journal of Modern Concepts in Material Science
Please follow the URL to access more information about this article
To know more about our Journals...Iris Publishers
To know about Open Access Publishers
No comments:
Post a Comment