Introduction
Emerging evidence suggests that elevated plasma levels of C-reactive protein (CRP) have become one of the strongest independent predictors in the development of atherosclerosis and coronary heart disease (CVD) [1]. CRP directly participates in the process of atherogenesis by modulating endothelial function and its concentration known to predict cardiovascular events [2]. Higher concentrations of CRP are associated with increased levels of serum triglycerides (TG) [3,4]. The CRP may, therefore, play a direct role in promoting the inflammatory component of atherosclerosis, and therapeutic CRP reduction might be a potential target for the treatment of atherosclerosis.
Elevated concentrations of plasma low density lipoprotein (LDL) cholesterol, low plasma high density lipoprotein (HDL) cholesterol and high plasma triglycerides (TG) or known as hypercholesterolemia are well- established risk factors for development of atherosclerosis and cardiovascular disease (CVD) [5]. Atherosclerosis, characterized by chronic inflammation in arteries, involves the accumulation of oxidized lipoproteins (OxLDL), an increased number of inflammatory cells (monocytes and lymphocytes), an infiltration of macrophages and degeneration of the arterial intima [6]. Accumulation of OxLDL and other substances, promotes the immune system cells [7] to migrate into the layers of the endothelium causes the breakdown of various substances and the attraction of LDL cholesterol particles to the site [8]. These LDL particles are further engulfed by monocytes, which then differentiate into macrophages (foam cells) leading to the formation of atherosclerotic lesions [9]. These lesions, known as atheromatous plaques, enlarge as cells and lipids accumulate in them, and begin to swell into the vessel lumen, consequent to the rupture of the plaque, thus causes lipid fragments and cellular debris released into the vessel lumen [10]. These particles are exposed to thrombogenic agents on the endothelial surface, resulting in the formation of a thrombus, or blood clot. If the thrombus is large enough to block circulation of coronary or cerebral blood vessels, this results in a heart attack or stroke [11]. Atherosclerosis complications often occur suddenly, and they can seriously jeopardize patients’ lives, and therefore any interventions aimed at reducing hypercholesterolemia are of great interest.
Tinospora crispa (L.) Hook f & Thomson, is a climber that can be found in primary rainforest of South East Asia including Malaysia, Indonesia, Thailand and Vietnam. Studies showed that the crude extracts and isolated compounds of T.crispa possessed a broad range of pharmacological activities such as cardioprotective, anti-diabetic, anti-inflammatory, antioxidant, immunomodulatory, cytotoxic and antimalarial activities [12]. Moreover, it was discovered that T. crispa possessed an anti- hypercholesterolemic activity and is beneficial in preventing the heart-related diseases. However, until to date there is insufficient literature documenting the effects of aqueous extract of Tinospora crispa on C-reactive protein and its anti- atherosclerotic effects in the event of hypercholesterolemia. Therefore, this study was aimed to measure the level of C-reactive protein as an inflammatory marker, continuing with a histological analysis of the aorta to further understand the possible mechanism contributing to the anti-atherosclerotic effects of tinospora crispa aqueous extract (TCAE).
Objectives
The objectives were to assess the role of CRP in the development of atherosclerosis and to assess the atherosclerotic plaque formation in hypercholesterolemic induced rabbits supplemented with TCAE.
Material and Methods
Collection of raw material and preparation of TCAE
About 10kg of fresh stem part of Tinospora crispa were collected from Forest Research Institute Malaysia (FRIM) at Kepong, Selangor. The plant was authenticated by FRIM botanist (Voucher number: SBID009/15). The stems were cleaned, washed, cut and dried using an oven dryer with operating temperatures of about 55°C. The weight of the samples was monitored every day until constant weight was obtained. Subsequently, the dried stems of the plant are ground to a particle size of about 1 to about 4 mm by using a 20 hp pilot scale grinder. The ground stem sample is kept at room temperature in a sealed environment prior to the extraction process.
The grounded stems of T. crispa were extracted with reverse osmosis water. The extraction processes used were 60° C temperature, 1:15 g/ml solvent to solid ratio and 1 hour of extraction time. The liquid extract then was filtered through Whatman No 1 filter paper (Whatman plc, Maidstone, UK). The filtrate was collected, and excess water was evaporated under reduced pressure using freeze dryer.
Animals and experimental procedures
Forty-two (42) healthy adult male New Zealand White rabbits (East Asia Rabbits, Malaysia) with initial mean weighting between 2.5 to 3.0 kg were used in the experiments. The animals were randomly houses in individual cages with free access to food and water with a regular light/dark cycle and under room temperature (28 ± 2°C, relative humidity 60-70 %) for 2 weeks for acclimatization before use. Following acclimatization, the animals were randomly segregated into six groups of seven rabbits each Table 1. The experiment was designed in order to evaluate the ability of TCAE at different concentrations, to prevent or delay the progression of atherosclerosis. Hence, the induction of experimental hypercholesterolemic rabbits were established by giving chow diet which were enriched with 0.5 % cholesterol for continuous 10 weeks to 5 groups of rabbits namely, group 1: Hypercholesterolemic-induced (H), group 2: Simvastatin control (SC), group 3: Low dosage of TCAE (200 mg/kg), group 4: Medium dosage of TCAE (450 mg/ kg), and group 5: High dosage of TCAE (600 mg/kg). In the Normal control (NC) group the rabbits were given with normal chow diet. Food and water were given ad libitum throughout the experiment. The STCAE and simvastatin were given via oral gavage while blood sampling was performed at week 0 and at week 10 of experimental period. The experimental protocol and animal handling throughout the study were in accordance with guidelines approved by the institution ethics committee with Certificate number: IACUC-FRIM/1 (2013)/07- 5.
C-Reactive protein
Human CRP was purchased from Sigma (solution in 0.02 mol/L Tris and 0.25 mol/L sodium chloride, pH 8.0). CRP was purified from human plasma by using Ca2+-dependent affinity of the protein to phosphorylcholine. Purity of the protein is ≥ 98 %, as determined by SDS-PAGE. The preparation displayed a single protein band of Mr ≈21 000. The physical state was examined by centrifuging 100 μg in 5 mL of a linear 10 % to 40 % (wt/vol) sucrose density gradient in 20 mmol/L Tris, 100 mmol/L NaCl, and 2 mmol/L Ca2+ buffer (50 000 rpm, vertical rotor VTi 65, 4 °C, 60 minutes, Beckman ultracentrifuge model L60). The protein sedimented in a symmetrical peak of ≈5.5S, and protein was not detected in higher Mr fractions (>19S). Thus, the CRP did not autoaggregate. During preparation, precautions were taken to avoid lipopolysaccharide contamination. The latter was excluded by Limulus endotoxin assay (Kinetic-QCL, BioWhittaker). Sensitivity of the assay is 0.015 to 400 IU/mL.
Histology study
Aorta preparation: At the end of the week 10, the rabbits were sacrificed. Midline thoracotomies were performed. Aorta tissue between its origin and bifurcation into the iliac arteries was taken gently, free of adhering tissues and washed with cold normal saline solution. The large part of origin was cut into 3 mm and was put into 10 % formalin for histology study (haematoxylin and eosin staining), whereas the ascending large part of aorta between its origin and bifurcation into the iliac arteries was opened longitudinally and prepared for plaque assay (Sudan IV staining).
Sudan IV Staining: Assessment of atherosclerotic plaque lesions: Atherosclerotic plaque areas were assessed by a previously described method. Briefly, the aortic strips were dissected from the ascending arch to the iliac bifurcation, and extraneous adipose tissue was removed. The aortas were opened longitudinally, rinsed several times with ice- cold saline and stretched onto a piece of cardboard. Then, it was fixed immersed in neutral 100 g/L buffered formalin solution for 24 h and then rinsed in 70 % alcohol. The tissue was then immersed in Herxheimer’s solution containing Sudan IV (5 g), ethyl alcohol (70 %, 500 mL) and acetone (500 mL) at room temperature for 15 min and washed in running water for 1 h. This staining allowed a clear depiction of the plaques due to their deep red colour.
Macroscopy study: The photographs of the intimal surface of the aorta were taken using digital camera (EOS Canon, Japan) and the intimal lipid lesions were determined quantitatively by estimation of the percentage of sudanophilic stained areas in the total aortic intimal area in photographs using Image Analysis Software. The total atherosclerotic area of the intimal surface of the aorta was measured in mm2. The extent of atherosclerosis was expressed as a percentage of the luminal surface that was covered by atherosclerotic plaques using the formula below:

Tissue blocking: An approximately 3mm of aortic arch were fixed in 10% formalin for a few days and prepared for light microscopy by dehydrating the tissue samples in an ascending series of alcohol dehydration, clearing with xylene and wax impregnation with paraffin wax for 14 h in an automatic tissue processor machine as shown in (Table 1).
Table 1: Tissue Dehydration in a Tissue Processor Machine (TP1020).
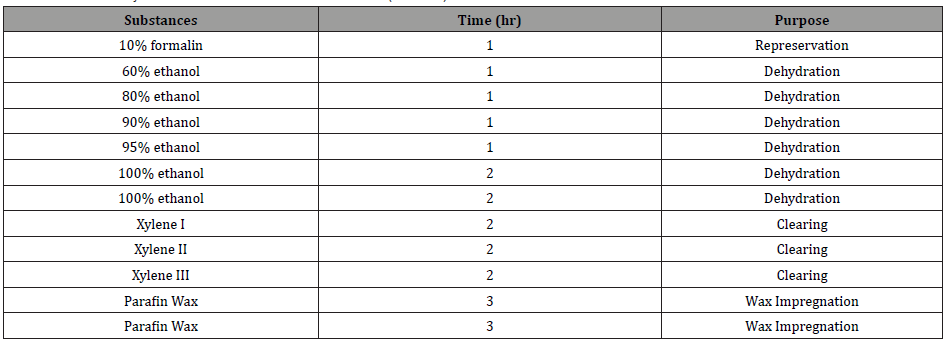
Tissue sectioning and staining: The tissues were embedded into block by paraffin wax at 62 °C and were cooled at 0 °C for 3 h to form solid block. This is followed by the sectioning process, whereas the tissues were trimmed and sectioned with the thickness of 4 to 5 μm ranges using a microtome machine. The tissues were then placed in the water bath, attached on glass slides and then were dried on a hot plate at 50 to 55 °C for 30 min and then kept at 37 °C. The tissues sections were then stained with Haematoxylin and Eosin (H&E) staining method using Autostainer Machine Table 2. The slides underwent processing, colorization and dehydration. After thoroughly dried from xylene, the slides were mounted with cover slips and mounted with DPX. The slides were then dried at room temperature for a few days before being analysed under light microscopy (Olympus CK2) (Table 2).
Table 2: Colouration with Haematoxylin and Eosin (H&E).
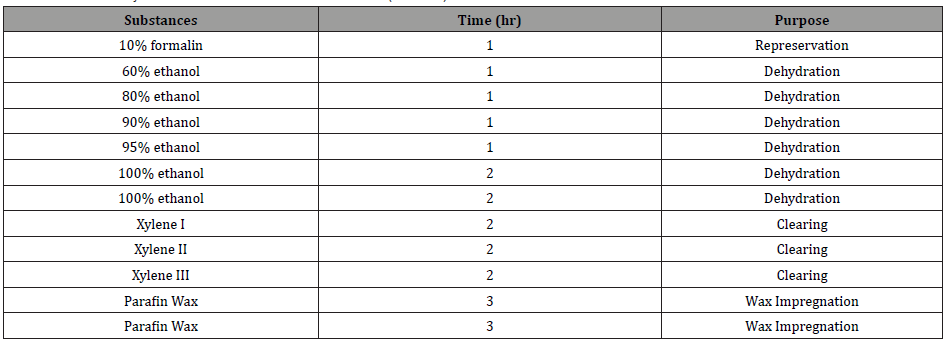
Table 3: Percentage of Atherosclerotic Plaques Coverage of All Groups.
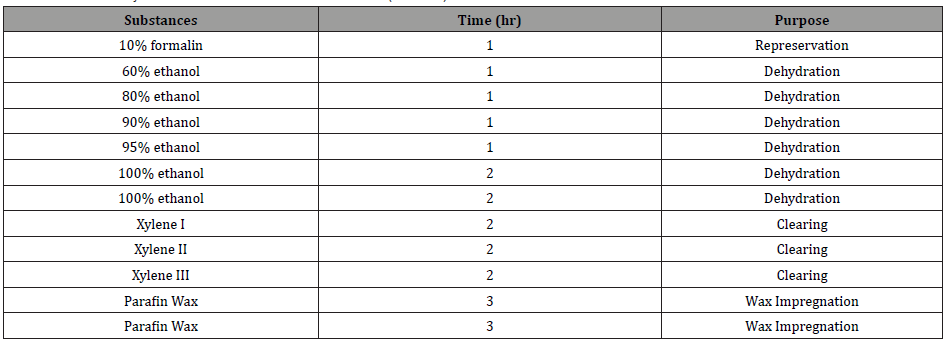
Notes: Each value represents the mean + SD. Values with the asterisk (*) are significantly different (p < 0.05) compared to hypercholesterolemic model group. Values with # are significantly different (p < 0.05) compared to normal control group. NC, normal control; H, high cholesterol diet group; TCAE, T. crispa aqueous extracts.
Quantitative analysis of histological data: For each slide of aorta, the plaque accumulation was analysed for the average determination of the thickness of the foam cells by using an image analysis system consisted of a Macintosh lix computer (Apple) equipped with a Frame Gabber Card (Quick capture, data translation), a Sony high-resolution video camera and a Trinitron Super Mac 21 in. color monitor.
Statistical analysis
All data were expressed as mean ± standard deviation (SD). One way ANOVA was used to compare the statistical differences between the treatment groups and the control group using SPSS version 21. Turkey post-hoc test was used for multiple group comparison analysis. Significant different was set at p<0.05.
Results
C-Reactive protein
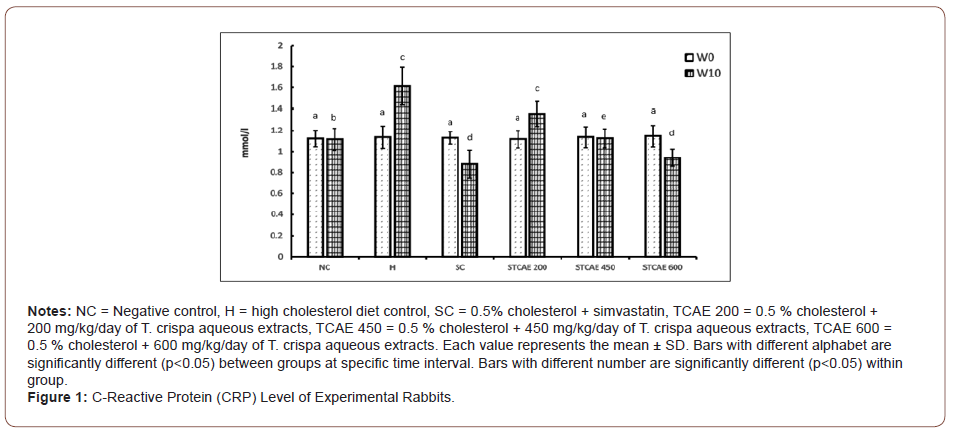
Figure 1 shows the results of CRP in all groups throughout the experimental period. At the beginning of the study period, there was no significant difference of CRP levels observed among all groups. However, feeding 0.5 % high cholesterol diet alone to the rabbits caused a significant increase (p<0.05) in the CRP levels at week 10 (1.61 mg/ml) compared to week 0 (1.14 mg/ml). In contrast to the H group, SC group showed a significant reduction (p<0.05) of CRP concentration throughout the experiment conducted with the CRP value 1.13 mg/L at week 0 and 0.88 mg/L week 10, respectively. Result also revealed that, the CRP level in rabbits supplemented with 200 mg/kg of STCAE was significantly increased (p<0.05) from week 0 to week 10 with the CRP value of 1.12 and 1.35 mg/L, respectively. On the other hand, it was observed that there was no significant different of the CRP concentration in rabbits supplemented with 450 mg/kg of STCAE from week 0 (1.14 mg/L) to week 10 (1.13 mg/L), respectively, in which the CRP level was significantly decrease (p<0.05) when compared to H group. Whereas, the CRP level in rabbits supplemented with 600mg/L at week 10 was significantly reduced (p<0.05) compared to week 0 and H group with the CRP concentration of 0.94 mg/L and 1.15 mg/L, respectively. Meanwhile, there was no significant difference observed in CRP level throughout the experiment conducted in the NC group (Figure 1).
Sudan IV staining of aorta
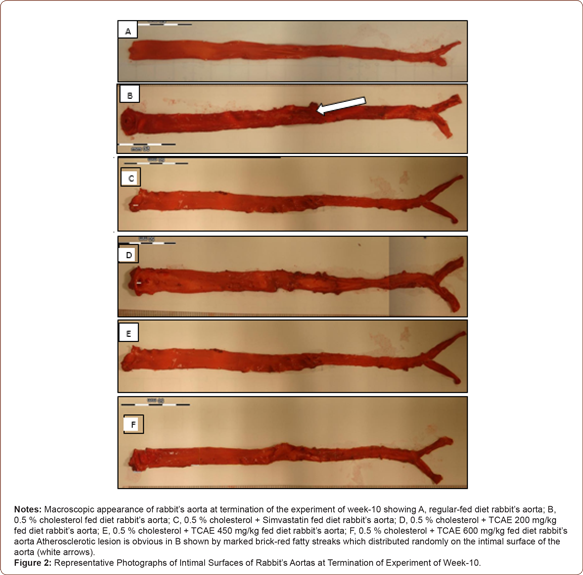
Photomicrograph of aorta with H&E staining
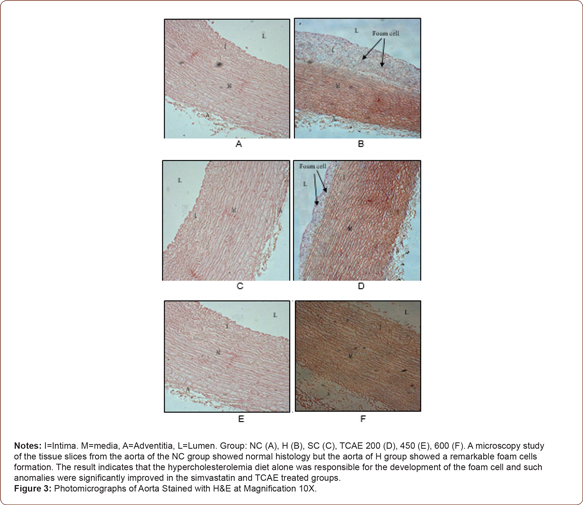
Effect of TCAE on atherosclerotic plaques coverage
Percentage of atherosclerotic plaques coverage for each treatment groups are shown in Table 3. Atherosclerotic plaques coverage of rabbits fed with the normal diet and rabbits supplemented with simvastatin and different concentration of TCAE measured using NC as a baseline. It was observed that almost no visible atherosclerotic plaques or lesion area in aorta of rabbits in the normal group. High cholesterol diet group showed marked increase of plaques coverage with 31.12 ± 2.18 % of coverage. Rabbits supplemented with 200, 450 and 600 mg/kg of TCAE showed marked reduction of atherosclerotic plaques coverage with 18.32, 50.87 and 66.25 % reduction respectively, when compared to hypercholesterolemic rabbit. Simvastatin reduced plaques coverage to a greater extent with 92.80 % of reduction (Table 3).
Effect of TCAE on thick of foam cells
The aorta’s atherosclerosis extension was further observed on its area of fatty region by using the detection of the formation of foam cells in the atherosclerotic lesions. Result found that there was no visible of foam cells observed in the thoracic aorta of rabbits fed with the normal diet and rabbits supplemented with simvastatin, 450 and 600 mg/kg of TCAE. The foam cells formation appeared much more severe in rabbits supplemented with high cholesterol diet than in rabbits supplemented with 200 mg/kg of TCAE. The thickness of tunica intima in the high cholesterol diet group and in the group supplemented with 200 mg/kg of TCAE were 186.50 ± 9.69 μm and 69.92 ± 4.82 μm respectively with significance different at p<0.05 (Figure 4).
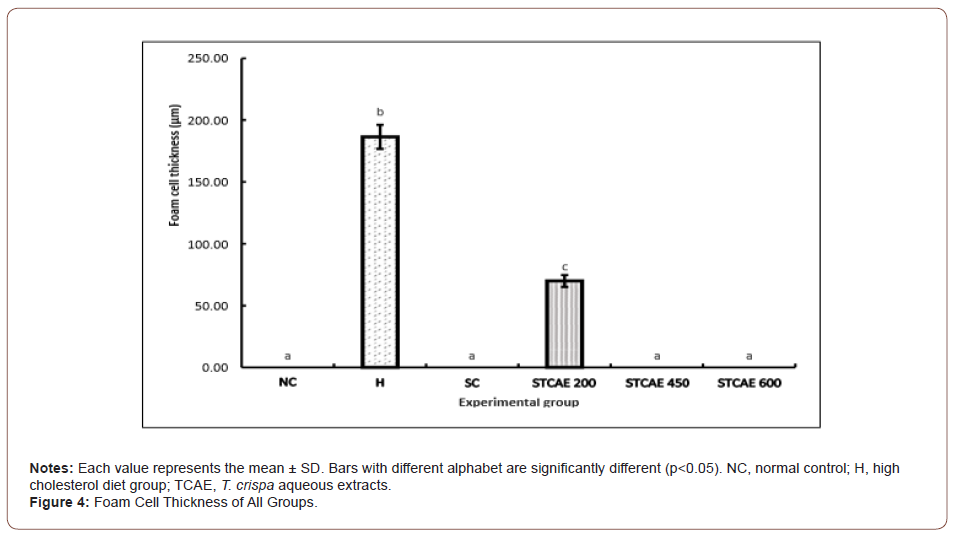
Discussion
Dietary cholesterol has been reported to contribute significantly elevation of plasma cholesterol. Increased cholesterol levels in the diet has been reported to elevate serum and aortic tissue cholesterol which subsequently increase aortic atherosclerotic [13] due to endothelial dysfunction [14].
The genesis of atherosclerotic associated with an increase level in markers of inflammation such as CRP and characterized by inflammation of the endothelium of the coronary arteries [15]. Evaluation on the CRP levels could provide a rapid indicator for determining individual risk factors of atherosclerosis in which individuals with elevated levels of CRP have a risk about 2 to 3 times higher than the risk of those with low CRP levels [16].
From the result obtained, it revealed that feeding high cholesterol diet to the rabbits for 10 weeks resulted in a significant increase of CRP levels compared to rabbits in the normal control group, indicating the incidence of early progression of atherosclerosis. The finding was supported by Yu, et al. [17] who reported that the plasma CRP levels were significantly higher in the cholesterol- fed rabbits than those of the control rabbits after 28 weeks of experiment. Similar finding was reported by Sun, et al. [18] who stated that the elevated CRP levels in plasma were correlated with the severity of atherosclerosis and hypercholesterolemia in rabbits. The excess of LDL in the circulation in the hypercholesterolemic- induced rabbits will cause more LDL to be accumulated in the sub-endothelial matrix which may undergo oxidation [8,19], later mediates OxLDL uptake by macrophage [20]. According to Mehta, et al. [21], CRP reduces the expression of nitric oxide synthase and prostacyclin synthase, binds with LDL and promotes its uptake by macrophages thus caused inflammation of the endothelium during early development stage of atherosclerotic process. In addition, accumulation of inflammatory cells, especially macrophages can produce a variety of cytokines that may stimulate the hepatic expression of the CRP gene and CRP production by the liver [18].
In contrast, supplementation of simvastatin to the hypercholesterolemic- induced rabbits significantly decreased (p<0.05) the CRP levels when compared to rabbits feeding with high cholesterol diet alone. Similar finding was reported by Shrivastava, et al. [15], who found that statin reduces CRP levels in many patients primarily by lowering the LDL cholesterol. The reduction of LDL levels in the circulation means less substrate available to be oxidized to produce oxidized- LDL (oxLDL) [22]. The less oxLDL in the intima caused the reduction of CRP levels resulting less formation of atherosclerotic plaque in the aorta [3].
Meanwhile, it was observed that supplementation of different concentration of TCAE to the hypercholesterolemic-induced rabbits were able to decrease the CRP levels in dose dependent manner as compared to the group of rabbit fed with high cholesterol diet alone. Interestingly, no significant different in the CRP concentration observed between the groups supplemented with 600 mg/ kg of TCAE and the group supplemented with simvastatin. TCAE at 600 mg/kg and simvastatin reduce 41.7 and 45.4 % of CRP concentration respectively, compared to hypercholestrolemic group. These results demonstrated the beneficial effect of supplemen tation with TCAE in inhibiting the production of CRP and subsequently reducing the extent of atherosclerosis, therefore, provide evidence that TCAE may have anti-inflammatory effects in addition to its lipid-lowering effects.
According to Dave, et al. [23], plaque stabilization and plaque regression were considered among the current approaches in managing atherosclerosis. Plaque stabilizations focus on stabilizing the content of plaque and strengthening the overlying endothelium whereas plaque regression focuses on the overall reduction in plaque volume and to reverse the arterial endothelium to its normal functional state.
The basic idea of plaque stabilization is to make the plaques more fibrous and reduce their lipid content with interventions such as lipid-lowering, anti-thrombotic, and anti-coagulant agents [24]. Decreased macrophage counts and MMP expression, and increased interstitial collagen content had led to increase the plaque stability [25]. Plaque regression on the other hand, emphasizes on longterm therapy in removing lipids and necrotic material, restoring normal endothelial function and repair of uncovered areas to bring about changes beyond just stabilization of vulnerable plaques [23].
In the present study, histological examination of tissues from hypercholesterolemic-induced-rabbits after 10 weeks of study period showed a morphological change in the aorta indicating that daily intake of high cholesterol diets causes development of early atherosclerosis lesions which consistent with the previous reports [13,26]. It was revealed that, the percentage of atherosclerotic plaques in rabbits fed with high cholesterol diet were significantly higher (p<0.05) compared to rabbits in any other groups. Similar findings were reported by Yanni [27], who found that administration of 0.5 %, w/w amount of cholesterol diet to the rabbits initiated the development of early atherosclerosis lesions similar to those in humans. According to Zulkhairi, et al., atherosclerosis occurred concomitant with an increase of MDA level in serum and aorta, indicating an increase in oxygen radical. Increase of oxygen radicals level can contribute to the high incident of endothelial injury which represents a critical initiating event in the development of atherosclerosis [28].
Interestingly, it was observed that the morphological changes of the aorta can be reversed by oral administration of TCAE. The results obtained in the present study showed that, supplementation of different concentration of TCAE were able to reduce significantly (p<0.05) the atherosclerotic plaque in dose dependent manner with 18.32, 50.87 and 66.25 % reduction respectively, compared with rabbits fed with high cholesterol diet, indicating the plaque regression ability of TCAE in inhibiting the atherosclerotic plaque progression and restoring normal endothelial function in the aorta.
Meanwhile, supplementation of 1.2 mg/kg/day simvastatin to the hypercholesterolemic-induced rabbits was able to reduce the lowest percentages of atherosclerotic plaque when compared with rabbits fed with high cholesterol diet. This indicates that simvastatin, which are already in clinical use to reduce cholesterol levels, are indeed effective in atherosclerotic patients. Studies carried out by Kano, et al. [29] and Soma, et al. [30], demonstrated statins reverse or impede the progression of atherosclerosis in rabbits. Whereas, a study by Gaist, et al. [31] revealed that statins show a good safety profile in patients with high cholesterol levels and cardiovascular disease, however, statins may be potentially associated with development of many side effects including hepatic damage, myalgias or polyneuropathy [32].
The results were then supported with evidence of severe thickness formation of foam cells in the thoracic aorta of rabbits fed with high cholesterol diets following 10 weeks experimental period. It was observed that the thickness of foam cells in rabbits fed with high cholesterol diet was significantly higher (p<0.05) compared to any other group, in line with the previous reports [33].
Meanwhile, the data from histopathological examination of simvastatin treatment fed rabbits revealed the absence of foam cells comparable with rabbits fed with the basal diet. The findings was supported by Libby [34] and Sukhova, et al. [35] who reported that statin reduced significantly macrophage content, adhesion molecules, cytokines and tissue factor expression in the lesions, as compared with dietary intervention alone. Clinical studies of plaque stabilization therapy by statin have consistently demonstrated increase in fibrous tissue content and reductions in the plaque lipid pool, however statin showed only modest reductions in plaque volume [36].
On the other hand, result showed that the thickness of foam cells in hypercholesterolemic-induced rabbits supplemented with 200 mg/kg of TCAE was significantly lower (p<0.05) with the reduction of 65.52 % as compared to the group without supplementation. Remarkably, there were no presence of foam cells observed in the thoracic aorta of rabbits fed with the 450 and 600 mg/kg of TCAE respectively, comparable with rabbits in the normal control and in the simvastatin groups, indicating the plaque stabilization ability of TCAE in stabilizing the content of plaque by reducing its foam cell and improving the endothelial functional. Yu, et al. [17] reported, reduction in macrophage and increase in smooth muscle cells in advanced lesions may affect plaque stability in rabbits.
The finding was in accordance to Zulkhairi, et al. who found that supplementation of 450 mg/kg T.crispa water extract exhibited no presence of foam cells in aorta of rabbit fed with high cholesterol diet. The ability of this plant in attenuating aortic fatty streak development could be associated to its lipid lowering activity [37,38]. It was supported by Stanely, et al. [39], who stated that administration of an extract of T. cordifolia roots for 6 weeks resulted in a significant reduction in serum and tissue cholesterol, phospholipids and free fatty acids in alloxan diabetic rats. On the other hand, according to Kamarazaman, et al. [40], T. crispa inhibits the formation of macrophage foam cells by preventing the survival and differentiation of monocyte into macrophage, thus reduce the risk of atherosclerosis-related diseases.
The development of atherosclerosis has been associated with the oxidative modification of LDL [17]. Therefore, the numerous and abundant antioxidant components in T. cripsa including phenolics [3] and flavonoids namely catechin, luteolin, morin and rutin. Studies have shown that the consumption of flavonoid antioxidant is inversely related to the risk of developing coronary heart disease. Flavonoids reduce LDL oxidation and also inhibit the aggregation and adhesion of platelets in the blood [41].
Other than oxidation of LDL, atherosclerosis also associated with inflammation in the intima of arteries [42]. It was reported that, expression of adhesion molecules such as vascular adhesion molecule 1 (VCAM-1) and intercellular adhesion molecule-1 (ICAM-1) promote the recruitment of leucocytes to the site of injury and later contribute in the development of the pro-inflammatory state and atherosclerotic lesion [43,44]. The macrophage colonystimulating factor (MCSF) will promote the transition of monocytes to macrophages and foam cells [9]. Those adhesion molecules can be stimulated by pro-inflammatory agents including cytokine Tumor Necrosis Factor (TNF)-α and oxidative stress along with several well-established risk factors such as hypercholesterolemia, hyperglycemia and hypertension which play important roles in the pathogenesis of coronary artery disease [45]. It is therefore believable that suppression of the pro-inflammatory endothelial cell state will limit the atherosclerosis process thus benefited in inhibiting the development of atherosclerotic lesions [46,47].
Previous in vitro study by Kamarazaman, et al. [48] exhibited that, supplementation of T. crispa aqueous and methanol extracts significantly reduced (p<0.05) secretion of ICAM-1, VCAM-1 and M-CSF, while in contrast significantly increased (p<0.05) secretion of nitric oxide (NO) in TNF-α induced HUVEC compared to untreated HUVECs. Increase in NO secretion could promote vascular vasodilatory effect thus preventing from the development of atherosclerotic plaque. NO is a potent endogenous vasodilator which plays a pivotal role in vascular homeostasis [49]. A reduction in NO synthesis may contribute to the initiation and progressivity of atherosclerosis [50,51].
The protective effect of T. crispa from the secretion of adhesion molecules possibly contributed by the presence of syringin. According to Rao, et al. [52], the anti-inflammation mechanism of syringin associated with lowering TNF-α, iNOS, ICAM-1 and its’ mRNA expression which later showed improvement on the injury of the organs. The finding was supported by Wua, et al. [53] who demonstrated that, syringin reduces inflammation response of TNF-α thus protects the target organs by lowering the inflammatory factors’ expression in the heart, brain and kidney comparable with Benazepril; an antihypertensive drug commonly used in clinic [54].
According to Pandey and Rizvi [55] and Opie & Lecour [56-60], a significant mechanism to prevent the development of atherosclerosis is to reduce cholesterol levels, reduce the oxidation of LDL, protect the endothelium and repress the synthesis of proinflammatory cytokines and adhesion molecules. Improvement in lipid profiles and cholesterol levels, reductions in LDL oxidation and protection from inflammatory factors as well improvement on the injury of endothelium have all been demonstrated with the intake of TCAE. However, further investigations are necessary required to further understand the exact mechanism of action of this compound as well as T. crispa as an anti-atherosclerotic agent.
Conclusion
Supplementation of TCAE was found to inhibit the progression of atherosclerotic plaque development induced by dietary cholesterol. The anti- atherosclerosis potential of TCAE was demonstrated by its ability in reducing the CRP levels, coverage area of atherosclerotic plaque and thickness of foam cells in the atherosclerotic lesions and preserves endothelial healing following arterial injury. Supplementation of 450 mg/kg of T. crispa extract was found to be the optimal concentration to be utilised towards the formulation of product for preventing hypercholesterolemia, atherosclerosis and reducing risk factors for coronary artery disease in the future.
To read more about this article...Open access Journal of Biology & Life Sciences
Please follow the URL to access more information about this article
https://irispublishers.com/sjbls/fulltext/the-effects-of-tinospora-crispa-aqueous-extract-on-c-reactive-protein-level-and-development-of-atherosclerotic-plaques.ID.000526.php
To know more about our Journals...Iris Publishers