Authored by Ebtsam M El Kady*,
Abstract
Polysaccharides (PSs) may be regarded as key ingredients for the production of bio-based materials in life sciences (medical devices, pharmaceutics, food and cosmetics). There are an enormous variety of polysaccharides that can be synthesized and/or released by marine algae. Both these marine organisms are excellent sources of PSs, most of them sulfated (S-PSs). Although some similarities may be found between the PSs from each group of organisms, they can be very heterogeneous and structurally different. The biological source and biodegradability of these biopolymers, coupled to the large variety of functionalities they encompass, make them promising compounds for the application in pharmaceuticals, therapeutics, and regenerative medicine. Some of the beneficial bioactivities demonstrated by the crude PSs and their derivatives, either in-vitro or in-vivo, include anticoagulant and/or antithrombotic properties, immunomodulatory ability, anti-tumor and cancer preventive activity. They are also good antilipidemic and hypoglycemic agents, and can be powerful antioxidants, antibiotics and anti-inflammatory. Other biomedical properties of PSs have been discussed, such as antinociceptive, angiogenic, cardioprotective, gastroprotective and hepatoprotective activities. The biomedical applications and potentialities of PSs in this area were listed, such as healing wound agents, mucobioadhesives for bone and soft tissue closure, bio lubricants to mitigate joint disorders caused by arthritis, vaccines for cancer immunotherapy, or in a new generation of bio textiles and medical fibers, in drug delivery systems, and scaffolds in regenerative medicine. From the extensive list above, the importance of this type of PSs from algae for medical use is quite obvious. However, despite all the interesting properties and potentialities for human health, the use of these PSs, especially those from algae need to be further explored. In particular, the toxicity and bioavailability of some of these polymers are yet to be studied on humans.
Background
Polysaccharides (PSs) are a high molecular weight polymer, consisting of at least ten monosaccharides mutually joined by glyosidic linkages. The glycosyl moiety of hemiacetal or hemiketal, together with the OH group of another sugar unit, formed the glyosidic linkages [1]. Unlike protein and nucleic acid, the structure of PSs is far more complicated based on the differences in (i) composition of monosaccharide residues, (ii) glyosidic linkages, (iii) sequence of sugar units, (iv) degrees of polymerization, and (v) branching point. Apart from those, other factors, such as differences of cultivars, origins, and batches, or even extraction methods and fraction procedures are evidenced to have significant influence on the physicochemical and structural properties of PSs. Owing to the rapid development of modern analytical techniques; the identification of PSs structures is becoming more and more feasible and convenient [1]. In recent years, researches have confirmed that PSs from natural products possess wide-ranging beneficial therapeutic effects and health-promoting properties. Specifically, seaweed derived PSs, such as alginate, fucoidan, carrageenan, laminaran, and agar [2], are widely distributed in biomedical and biological applications [3-7], for example, tissue engineering, drug delivery, wound healing, and biosensor due to their biocompatibility and availability.
Fungal PSs, derived from Grifola frondosa, Lentinula edodes, oyster mushroom, as well as Ganoderma, Flammulina, Cordyceps, Coriolus, and Pleurotus, and so forth, are demonstrated to have multiple bioactivities [8-11], including immunomodulating, anticancer, antimicrobial, hypocholesterolemic, and hypoglycemic effects. Bacterial extracellular PSs, loosely associated with bacterium, capsular PSs, tightly bound to bacteria surface, and lipopolysaccharides, always anchored to cell surface by lipid, are nontoxic natural biopolymers and provide extensive applications in areas such as pharmacology, nutraceutical, functional food, cosmeceutical, herbicides, and insecticides [12].
If PSs contains only one kind of monosaccharide molecule, it is known as a homopolysaccharide, or homoglycan, whereas those containing more than one kind of monosaccharide are heteropolysaccharides. The most common constituent of PSs is glucose, but fructose, galactose, galactose, mannose, arabinose, and xylose are also frequent [13,14]. PSs are structurally diverse classes of macromolecules able to offer the highest capacity for carrying biological information due to a high potential for structural variability [15]. Whereas the nucleotides and amino acids in nucleic acids and proteins effectively, interconnect in only one way, the monosaccharide units in PSs can interconnect at several points to form a wide variety of branched or linear structures [16]. This high potential for structural variability in PSs gives the necessary flexibility to the precise regulatory mechanisms of various cell-cell interactions in higher organisms. The PSs of mushrooms occurs mostly as glucans. Some of which are linked by β-(1---3), (1---6) glycosidic bonds and α-(1---3)-glycosidic bonds but many are true heteroglycans. Most often there is a main chain, which is either β-(1---3), β-(1---4) or mixed β-(1---3), β-(1---4) with β-(1---6) side chains. Hetero-β-D-glucans, which are linear polymers of glucose with other D-monosaccharides, can have anticancer activity but α-D-glucans from mushroom usually lack anticancer activity [15]. Heteroglucan side chains contain glucuronic acid, galactose, mannose, arabinose or xylose as a main component or in different combinations
The number of potential PSs structures is almost limitless but in practice many such polymers are unlikely to possess useful physical properties. Even now it is difficult to relate the chemical structure elucidated for any specific PSs to its physical functionality [17]. Currently only a small number of biopolymers are produced commercially on a large scale. However, this limited group of products exhibits an extensive range of physical properties and also provides several models for study by microbiologists, carbohydrate and physical chemists and molecular biologists.
PSs widely exist in the animals, plants, algae and microorganism. Together with proteins and polynucleotides, they are essential biomacromolecules in the life activities and play important roles in cell–cell communication, cell adhesion, and molecular recognition in the immune system [18]. In recent years, some bioactive PSs isolated from natural sources have attracted much attention in the field of biochemistry and pharmacology. They exhibit various biological activities affected by different chemical structures. Suarez et al. [19] reported that the immunostimulatory activity of arabinogalactans extracted from Chlorella pyrenoidosa cells depended on their molecular weights. The higher molecular weight arabinogalactans exhibited immunostimulatory activity, but the lower molecular weight fractions did not. Further researches show that the activities of PSs are not only dependent on their chemical structures, but also are related to their chain conformations [20]. It is known that the anti-tumor activities may be related to the triple helical conformation of the β-D-(1---3)-glucan backbone chain for some PSs, such as lentinan from Lentinus edodes [19,21] and schphylizophyllan from Schizophyllum commune [22,23]. In view of the fact that, Sakurai and Shinkai [24] were the first to find that schizophyllan may form a helical complex with single stranded homopolynucleotides, many works about preparing a complex of schizophyllan and DNA or RNA for a nontoxic gene delivery system have been developed [25-32]. Generally, it is interesting and important to elucidate the relation among chemical structures, chain conformations of PSs and their biological activities. However, PSs are usually composed of various monosaccharides linked with different glucoside bonds. Some PSs has hyperbranched structures. Moreover, PSs often has high molecular weights, and tends to form aggregates in solution that can mask the behavior of individual macromolecules. In consequence, to characterize the chemical structures and chain conformations of PSs is not an easy task. This article attempts to review the current structural and conformational characterization of some importantly bioactive PS isolated from natural sources (Figure 1).

The chemical structures were analyzed by FTIR, NMR, GC, GC– Mass and HPLC. The chain conformations of PSs in solutions were investigated using static and dynamic light scattering.
PSs already proved to have several important properties [33- 43]. However, the attempts to establish a relationship between the structures of the PS and their bioactivities/actions have been a challenge due to the complexity of this type of polymers. In fact, aside from the homogalactan from Gyrodinium impudicum [44], the β-glucan from Chlorella vulgaris [45] and the PSs from a few species of algae, most of these carbohydrates are highly branched hetero polymers with different substituents in the various carbons of their backbone and side sugar components. Additionally, the monosaccharide composition and distribution within the molecule, and the glyosidic bonds between monosaccharides can be very heterogeneous, which is a real impairment for the study of their structures. Moreover, this heterogeneity also depends on the species, between strains of the same species, and on the time and place of harvest. Nevertheless, there are always some similarities between the PSs from each group of seaweeds: often, fucoidans are extracted from brown algal species, agaroids and carrageenans come from red algae, and ulvans are obtained from green algae. Regarding cyanobacteria and as far as we know, there are not common names for their PSs, to the exception of spirulan from Arthrospira platensis. There are species that, besides producing large amounts of these useful polymers, they secrete them out into the culture medium and these polymers are easily extracted [38]. Both algae are cyanobacteria excellent sources of PSs, most of them being sulfated (S-PSs). They are associated with several biological activities and potential health benefits, making them interesting compounds for the application in pharmaceuticals, therapeutics, and regenerative medicine. Some of the beneficial bioactivities demonstrated by the crude PSs and their derivatives, either in-vitro or in-viv, upon various kinds of cell-lines and animal models, include anticoagulant and/or antithrombotic properties, immunomodulatory ability, anti-tumor and cancer preventive activity. They are also good antidislipidaemic and hypoglycaemic agents, and can be powerful antioxidants, antibiotics and antiinflammatory. The S-PSs from Enteromorpha and Porphyridium have demonstrated strong antitumor and immunomodulating properties [46-48] those from Caulerpa cupressoides and Dyctiota menstrualis are good antinociceptive agents [49,50], and the S-PSs from Cladosiphon okaramanus showed angiogenic, gastro- and cardioprotective bioactivities [33,51,52].
Some Structural Characteristics of Polysaccharides from Algae
The chemical structure of PSs from algae may significantly determine their properties, namely physico-chemical and biochemical, and reflect their physical behavior and biological activities.
Macroalgae
Macroalgae who’s PSs have been studied more often; belong to the group’s brown algae (Phaeophyceae) green algae (Chlorophyta) and red macroalgae (Rhodophyta). Brown algae usually contain fucoidans; the oligosaccharides obtained from the hydrolysis of fucoidans may often contain galactose, glucose, uronic acids, and/or other monosaccharides, linked together and to the main chain by different types of glycosidic bonds. This is the case, for example, for the laminaran from E. bicyclis, or the galactofucan from Sargassum sp., and the fucan from P. tetrastromatica. However, the structure complexity of these fucoidans makes difficult to establish a relationship between the PS-chains/composition and their biological actions, and/or some kind of protocols to design universal pharmaceuticals or other drug-like substances to prevent and/ or cure specific diseases [53]. The monosaccharide composition, the linkage types, the overall structure of fucoidans, and some of their di- and oligosaccharides were well explored by Li et al. [54], Ale et al. [55] and Fedorov et al. [35]. Ale et al. [55] showed the difference between S-PSs from three species of Fucus by focusing on the various substituents at C-2 and C-4 carbons, despite the similarities of their backbones; they also highlighted the possible structures of fucoidans from two species of Sargassum [56,57]. Among them are the schemes for the components of the main chain showing either the (1---3)-, and (1---3)- and (1---4)-linked fucose residues or some di- and trisaccharide repeating units for A. nodosum, C. okamuranus, L. saccharina and some species of Fucus. On the other hand, Fedorov et al. [35] focused on the structures and bioactivities of different S-PSs, such as galactofucan from Laminaria and laminarans from E. bicyclis. Red algae contain large amounts of S-PSs, mostly agaroids and carrageenans, with alternating repeating units of α-(1---3)-galactose and β-D-(1---4)-galactose [58], and/or (3---6)-anhydrogalactose [59]. Substituents can be other monosaccharides (mannose, xylose), sulfate, methoxy and/or pyruvate groups and the pattern of sulfation dividing carrageenans into different families, for example, in C-4 for κ-carrageenan, and in C-2 for λ-carrageenan. In addition, the rotation of galactose in 1,3-linked residues divides agaroids from carrageenans [60]. Apart from agarans [60], found in species of Porphyra, Polysiphonia, Acanthophora, Goiopeltis, Bostrychia or Cryptopleura are also good sources of κ-carrageenan (E. spinosa and K. alvarezii), λ-carrageenan (Chondrus sp, G. skottsbergii and Phillophora) [61], I-carrageenan (E. spinosa) [62], and other heterogalactans with mannose and/ or xylose building up their backbones. Among these, we may find xylogalactans in N. fastigiata and xylomannans in S. polydactyla [63,64].
Regarding green algae, the information on their structures and applications is scarce. Wangs et al. [41] has made an excellent overview on those properties for the S-PSs from several genera of green algae. These S-PSs are very diverse and complex, with various types of glycosidic bonds between monomers, and include galactans (Caulerpa spp.), rhamnans (C. fulvescens and Enteromorpha), arabino- and pyruvylated galactans (Codium spp.), and the most known ulvans from Ulva spp and E. prolifera. Wang et al. [41] also included some repeating aldobiuronic di-units for the backbone of ulvans, containing aldobiouronic acid or glucouronic acid (U. armoricana and U. rigida, respectively), disaccharides sulfated xylose-sulfated-rhamnose, and a trisaccharide unit composed by 1,4-linked glucouronic acid, glucouronic acid and sulfatedrhamnose. The backbone of rhamnans seems to be somewhat simpler, but other types of glycosidic bonds can also appear. Four repeating disaccharide units were indicated for the homo polymer of M. latissimum [65]. Species from Codium are very interesting: their S-PSs may include different percentages of arabinose and galactose, giving place to arabinans (C. adhaerens [66], galactans (C. yezoense) [67], arabinogalactans [41]. Pyruvylated galactans were also identified in C. yezoense [67], C. isthmocladium [68] and C. fragile [69]. Some other species of Codium present other PS-types such as β-D-(1---4)-mannans in C. vermilara [70], or the rare β-D- (1---3)-mannans in C. fragile [71], with various sulfation patterns
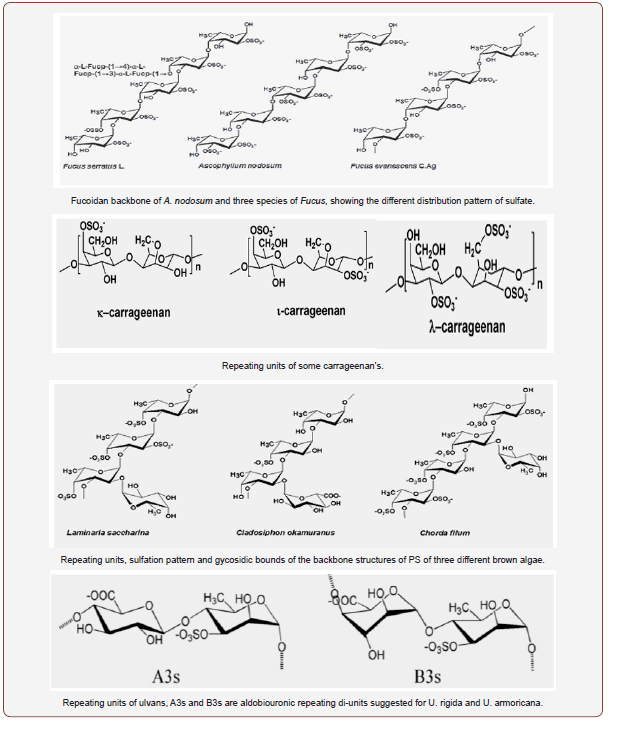
Cyanobacteria
The characteristics of the various PS produced by cyanobacteria, including their composition and structure, were recently discussed [72]. Some particular aspects about these polymers came to light. For example, it seems that concerning cyanobacteria only G. impudicum and C. vulgaris contain homopolysaccharides of galactose and glucose [44,45], respectively, while the PS from the other species are hetero polymers of galactose, xylose and glucose in different proportions. Rhamnose, fucose and fructose can also appear, and some of the PS presents uronic acids as well. The glycosidic bonds are described for only a few PS, such as the one from Aphanothece halophytica, whose monosaccharides are mainly 1,3-linked, but linkages of type 1 also appear for glucose and glucouronic acid [73], which suggests that these two last molecules are terminal, and some multiple bonds, such as 1,2,4-linked and 1,3,6-linked mannose residues [73], are present as well, suggesting some branches coming out from the backbone of the PS. Further, there are some special features of PS, as it is the case of acofriose 3-O-methyl-rhamnose in the polymers of Chlorella [74], Botryococcus braunii and calcium-spirulan (Ca-Sp) of Arthrospira platensis [75]. In Porphyridium cruentum, an aldobiuronic acid [3-O-(α-D-glucopyranosyluronic acid)-L-galactopyranose], and two hetero-oligosaccharides were also identified [76], and so did two other aldobiuronic acids [77] which were also found in other species of Porphyridium and Rhodella [19]. Furthermore, other repeating disaccharide units [78,79] and some oligosaccharides were also highlighted [79]. In addition, Ford and Percival [80] found that the structure of the S-PS from Phaeodactylum tricornutum was a ramified sulfated glucoronomannan, with a backbone composed by β-(1---3)-linked mannose; a triuronic acid, an aldobiuronic acid and a glucan made of β-(1---3)-linked glucose were also identified as being constituents of the side chains of that polymer

Potential Pharmaceutical Applications of Polysaccharides from Algae
The PSs are complex and heterogeneous macromolecules, coming from different genera belonging to the larger groups of algae, and species and strains of the same genus. Repeatedly, difficulties are found in identifying their chemical structure and therefore, their biological activities not being thoroughly understood. Few researchers have focused on such a challenging task as the exploitation of possible relation chemical structure activity of PSs. One approach to look for structure biological activity relationships has been to make inferences based on information obtained from studies of invertebrate sulfated polysaccharides that have a regular structure and, thus, could be more easily studied [60,81]. The types of glycosidic linkages and the contents and positions of the sulfate groups may be significantly different in the various S-PS, depending on species, region of the thallus, growing conditions, extraction procedures, and analytical methods [68,82]. The biological and pharmacological activities of S-PSs normally result from a complex interaction of several structural features, including the sulfation level, distribution of sulfate groups along the polysaccharide backbone, molecular weight, sugar residue composition, and stereochemistry [83,84]. For instance, the general structural features of fucans that are important in their anticoagulation activity include the sugar composition, molecular weight, sulfation level and the position of sulfate groups on the sugar backbone [85- 88]. Furthermore, it has been observed that the antiviral activity of S-PSs increases with the molecular weight [89]. Galactans, fucans and galactofucans are representative polysaccharides from brown and red algae that differ in structure, sulfation level and molecular weight, and yet all were shown to inhibit HSV-1 and HSV-2 infection [90]. Newly, by using NMR, it was found that branched fucoidan oligosaccharides might present higher imunoinflammatory activity than linear structures, because they were better at inhibiting the complement system [91]. Usov [58] compared two sulfated galactans from Botryocladia occidentalis and Gelidium crinale. He concluded that the interaction of the S-PSs with different compounds participating in the coagulation process depends on the differences in the structural features; unfortunately, data on the configuration of galactose in the galactan from G. crinale are not sufficient to fully understand the relationship.
Antiviral and antimicrobial activities
During the last decade, the number of antivirals approved for clinical use has been increased from 5 to more than 30 drugs [92]. However, as these drugs are not always efficacious or well tolerated and drug resistant virus strains are rapidly emerging, there is still a great demand for further drug development including novel modes of action. A very promising approach is the antiviral screening of products derived from natural sources, such as marine flora and fauna, bacteria, fungi, and higher plants. Among them, marine algae represent one of the richest sources of bioactive compounds, and algae-derived products are increasingly used in medical and biochemical research [93]. HIV-1 infection is a multistep process beginning with the attachment of the version to cell surfaces and entry into lymphoid target cells. In several cell types, including CD4+ helper T cells, macrophages, and dendritic cells [94,95], the virus can establish productive or persistent infection. During virus spread, HSV-1 virons are attached via their envelope gp120 glycoprotein to the CD4 surface protein of T-lymphocytes in conjunction with a chemokine (CXCR4 or CCR5) co receptor (Figure 2).
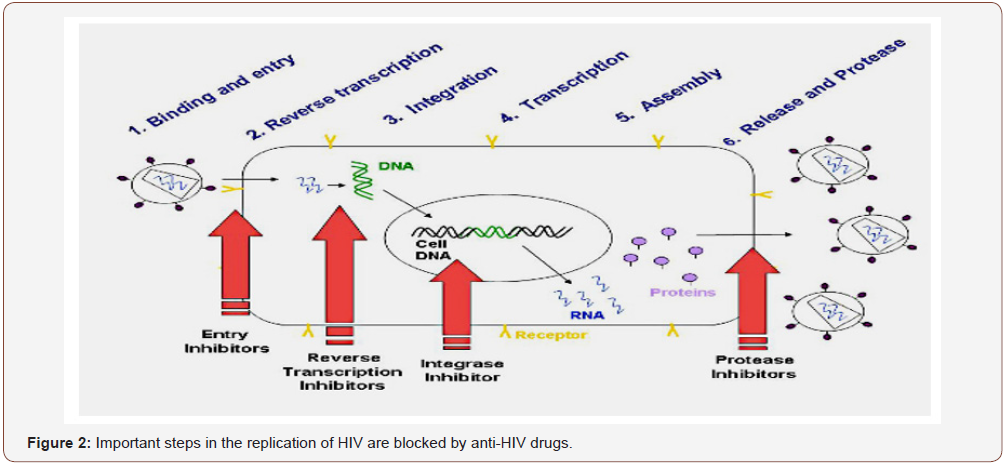
An overview on the antiviral activity against several kinds of virus and retrovirus, enveloped or naked was well documented by Carlucci et al. [96] & Wijesekara et al. [2]. These reviews focused on the HIV type 1 and type 2, the human papilloma virus (HPV), the encephalo-myocarditis virus, the hepatitis virus type A and type B and the dengue and yellow fever virus. The inhibition of infection by most of these viruses was explained by the action of S-PSs, which might block the attachment of visions to the host cell surfaces [97,98]. Another way of exerting their activity is by inhibiting the replication of the enveloped virus, such as the HIV, the human cytomegalovirus (HCMV) and the respiratory syncytial virus (RSV) [60,66,99], either by inhibiting the virus adsorption or the entry into the host cells. Some of the S-PSs are effective only if applied simultaneously with the virus or immediately after infection [60]. Another mechanism of action of fucoidans and other S-PSs is through the inhibition of the syncytium formation induced by viruses [2,100]. Some sulfated-xylomannans were reported to present antiviral sulfate-dependent activity, as it was the case of PSs from S. polydactyla and S. latifolium, which inhibited the multiplication of HSV-1 in Vero-cells [1,101]. Additionally, the molecular weight (MW) seems to play an important role in the antiviral properties of the S-PSs, the effect increasing with the molecular weight [60]. However, other structural features can be co-responsible for the reinforcement of the antiviral effectiveness, like sulfation patterns, composition and distribution of sugar residues along the backbone, and the complexity of the polymers [60,64,83,90]. Further, the fucoidans from L. japonica already proved their effectiveness in fighting both RNA and DNA viruses [54], such as poliovirus III, adenovirus III, ECHO6 virus, coxsackie B3 and A16 viruses. Moreover, these S-PSs can protect host cells by inhibiting the cytopathic activity of those viruses [102].
In addition to their virucidal activity against HIV and other viruses associated to sexually transmitted diseases (STD) [103], including HPV, some carrageenans might find application as vaginal lubricant gels and coatings of condoms, with microbicidal activity, for they do not present any significant anticoagulant properties or cytotoxicity [104,105]. Furthermore, some fucoidans, apart from inhibiting attachment of virus particles to host cells, were able to inhibit the attachment of human spermatozoids to the zona pellucida of oocytes [106]; this property could be used for the development of a contraceptive gel with microbicidal characteristics [40]. The PSs from some algae, and which may be released into the culture medium, showed antiviral activity against different kinds of viruses, such as the HIV-1, HSV-1 and HSV-2, VACV and Flu-A, as described by Raposo et al. [72] S-PSs, in particular, proved to increase the antiviral capacity [107]. In fact, the antiviral activity of the PSs may depend on the culture medium, algal strain and cell line used for testing, but also on the methodology, and the degree of sulfation, as is the case of PSs from P. cruentum [108,72]. Despite the slight toxicity that some PSs may present, they could be safely applied in in vivo experiments, decreasing the replication of the virus VACV, for instance [109]. The mechanisms involved in the antiviral activity of S-PSs may be understood analyzing what happens when cells are infected by a virus. Just before infection, viruses have to interact with some glycosaminoglycan receptors (GAG), such as heparin sulphate (HS) [110]. The GAG to which a protein can be covalently bound are part of the target cell surface and can also be found in the intracellular matrix of various connective and muscle tissues. S-PSs may impair the attachment of the virus particles by competing for those GAG-receptors, as they are chemically similar to HS [96,111], most of them having a covalently linked core protein [112,113].
Besides, as it happens with GAG, S-PSs are negatively charged and highly sulfated polymers [96,114,115], whose monosaccharide distribution pattern might influence the specificity of the bound protein, determining several biological functions [110]. For viruses to attach to the host cell surface, the linkage between the basic groups of the glycoproteins of the virus and the anionic components of the PSs (sulfate) at the cell surface must be established [83]. In fact, whichever the algal PSs are, either from algae, by mimicking this GAG, they may induce the formation of a virus-algal PSs complex, thus, impairing the cell infection by blocking the interaction virus-host cell receptor. Hidari et al. [114], for instance, showed that dengue virus (DENV) establishes an exclusive complex with fucoidan, and viral infection is, therefore, inhibited. They suggested that arginine-323 had a high influence on the interaction between the DENV-2 virus and the fucoidan, in an in-vitro experiment with BHK-21 cells. These researchers also found that glucuronic acid seems to be crucial since no antiviral activity was observed when this compound was reduced to glucose. Sulfated polysaccharides from algae, such as alginates, fucoidans and laminaran appear to have antibacterial activity against E. coli and species from Staphylococcus. A fucoidan from L. japonica and sodium alginate were found to inhibit E. coli [116], for example, by adhering to bacteria and killing those microorganisms [103], thus showing bactericidal properties. This type of PS is also a good antibacterial agent against Helicobacter pylori, eradicating their colonies, restoring the stomach mucosa, in clinical trial studies, and regenerating biocenosis in the intestines [117]. Laminaran from Fucus, Laminaria, A. nodosum and U. pinnatifida demonstrated to have an effect on pathogenic bacteria [118] as well, with the advantage of being unable to promote blood coagulation [119]. In contrast, the carrageenans from some seaweeds [120] and the S-PSs from the red algae Porphyridium cruentum, despite the higher concentration used [72], showed a significant inhibitory activity against S. enteritidis. In fact, some PSs from microalgae, such as A. platensis, may present antibacterial properties against some specific bacteria, the activity depending on the solvent used to extract the polymer [38]. By stimulating the production and/or expression of ILs, dectin-1 and toll-like receptors-2 on macrophages and dendritic cells, respectively, (1---3)-β-glucans from C. vulgaris, and laminarans, also induced antifungal and antibacterial responses in rats [121], and some resistance to mammal organisms towards infections by E. coli [122]. Therefore, these types of PSs promise to be good antimicrobial agents.
Anti-Inflammatory and immunomodulatory activities
PSs from algae have long demonstrated to have biological and pharmaceutical properties, such as anti-inflammatory and immunomodulation [81,123,72]. Nevertheless, the antiinflammatory properties may be shown in several ways, depending on the PSs, its source and type/site of inflammation. There is growing evidence that S-PSs are able to interfere with the migration of leukocytes to the sites of inflammation. For example, the heterofucan from D. menstrualis decreases inflammation by directly binding to the cell surface of leukocytes, especially polymorphonuclear cells (PMNs). It completely inhibits the migration of the leukocytes into the peritoneal cavity of mice where the injured tissue was after being submitted to simulated pain and inflammation, without the production of pro-inflammatory cytokines [49]. Every so often, the recruitment of these PMNs shows to be dependent on P- and/or L-selectins, as it was demonstrated for fucoidans of some brown algae [33,124]. Some other studies refer the association of the anti-inflammatory activity with the immunomodulatory ability. This seems to be the case in the work by Kang et al. [125] who simulated an inflammation process in RAW 264.7 cells induced by lipopolysaccharides (LPS). They found that the fucoidan from E. cava inhibited, in a dose-dependent manner, the enzyme nitric oxide synthase induced by LPS (iNOS) and the gene expression for the enzyme cyclooxygenase-2 (COX-2) and, as a consequence, the production of nitric oxide (NO) and prostaglandin E2 (PGL2). Li et al. [65] confirmed the anti-inflammation mechanism in vivo via the immunomodulatory system in-vivo, since the fucoidan from L. japonica reduced the inflammation of rats’ myocardium damaged cells, by inactivating the cytokines HMG B1 and NF-κB, two groups of proteins secreted by the immune cells during inflammatory diseases. These protective and regenerative effects of fucoidans, via the immunomodulatory system, were also verified in the destruction/proteolysis of connective tissue by Senni et al. [126]. These researchers referred to the fact that severe inflammation and the subsequent excessive release of cytokines and matrix proteinases could result in rheumatoid arthritis or chronic wounds and leg ulcers, which could be treated with fucoidans [126].
In addition to the SPs from Ulva rigida, green algae [127], the S-PSs p-KG03 from the marine dinoflagellate G. impudicum, also activates the production of nitric oxide and immunostimulates the production of cytokines in macrophages [128]. The enhancement of the immunomodulatory system by some S-PSs from marine algae is also a way for S-PSs to suppress tumour cells growth and their proliferation, and to be natural neoplastic-cell killers. Studies with arabinogalactan and other fucoidans revealed them to be immunostimulators by activating macrophages and lymphocytes, which suggests their effectiveness in the immuno-prevention of cancer [43,129]. The PSs from U. pinnatifida was also suggested to treat/relieve the symptoms of pulmonary allergic inflammation as it suppresses the activity of Th2 immune responses [130]. On the other hand, fucoidan activated macrophages and splenocytes to produce cytokines and chemokines [131]. PSs from algae, such as Porphyridium, Phaeodactylum, and C. stigmatophora, showed pharmacological properties, such as anti-inflammatory activity and as immunomodulatory agents, as reported by Raposo et al. [72]. Some of these S-PSs, for example, the ones from C. stigmatophora and P. tricornutum, have revealed anti-inflammatory efficacy in vivo and in vitro [132]. The mechanisms underlying the anti-inflammatory and immunomodulatory activities may be understood by making some considerations at the molecular level. On one side, the protein moiety that is covalently bound to most PSs seems to play a critical role in the activation of NF-κB and MAPK pathways involved in the macrophage stimulation [133,113]. This was evidenced in an in vitro experiment performed by Tabarsa et al. [113]. They showed that the PSs from C. fragile was not able to stimulate RAW264.7 cells to produce NO and the protein alone was also unable to induce NO release, but the complex S-PS-protein did inhibit the inflammatory process. On the other side, several other researchers found that proteins were not essential or responsible for the immunostimulatory responses of the cells [134,127]. Additionally, Tabarsa et al. [135] confirmed that the sulfate content and the MW were not crucial for the stimulation of murine macrophage cells. In fact, both desulfated and LMW-PS derivatives of C. fragile produced immunomodulatory responses similar to the ones of the original PSs. In contrast, the S-PSs from U. rigida induced a strong sulfatedependent release of NO [127], thus, the sulfate content showing to be essential for the stimulation of macrophages.
These researchers mentioned the possibility of the sulfate interfering in the interaction PS-cell surface receptors. The interaction of algal S-PSs with the complement system suggests that they might influence the innate immunity to reduce the proinflammatory state [91,81]. In addition, algal polysaccharides have been shown to regulate the innate immune response directly by binding to pattern recognition receptors (PRRs) [136]. For example, λ-carrageenan stimulated mouse T cell cultures in a tolllike receptor-4 (TLR4) [138]. Different effects were observed in other types of S-PSs: Zhou et al. [137] proved that carrageenans from Chondrus with LMW s better stimulated the immune system. The same trend was verified for the S-PSs from the red algae Porphyridium [139], a 6.53 kDa LMW-fragment at 100 μg/mL presenting the strongest immunostimulating activity. It is worth remarking that carrageenans from red seaweeds are recognized for triggering potent inflammatory and carcinogenic effects either in rats or mice cells [111]. However, while some carrageenans stimulate the activity of macrophages, others inhibit macrophage activities [2]. While PSs from various algae do not show anticoagulant and/or antithrombotic activities, attention should be paid to the anticoagulant properties of some PSs, since their use could cause severe bleeding complications.
Anti-proliferative, tumour suppressor, apoptotic and cytotoxicity activities
The current understanding of the anti-cancer and immunomodulating effects of PSs are as follows: (i) prevention of onset of cancer by oral consumption of mushrooms or their preparations; (ii) direct inhibition of growth of various types of cancer cells; (iii) immunostimulating activity against cancers in combination with chemotherapy; (iv) preventive effect on spreading or migration of cancer cells in the body [15]. On the whole, the indirect anti-cancer as well as immunostimulatory effects of lentinan is attributed to the activation of many immune cells. Lentinan can activate them to modulate the release cell signal messengers such as cytokines. The increases in cytokine production in immune cells have been studied in mice and in humans [140,141].
Because of the growing number of individuals suffering from different types of cancer and the secondary effects of synthetic chemicals and other types of treatment used against tumour damages, research was driven towards demand for natural therapeutics with bioactive compounds. In this context, S-PSs from both macro algae and micro algae already proved to have antitumor biological activities. A sulfated-fucoidan from C. okamuranus exhibited anti-proliferative activity in U937 cells by inducing cell apoptosis following a pathway dependent of Caspases-3 and -7 [142]. In another study, conducted by Heneji et al. [143], a similar fucoidan induced apoptosis in two different leukaemia cell lines. These results indicate that fucoidans might be good candidates for alternative therapeutics in treating adult T-cell leukaemia [43]. Sulfated-fucoidans from E. cava also seem to be promising to treat other types of human leukaemia cell-lines [144]. There was some evidence that the fucoidan from L. guryanovae inactivated the epidermal growth factor (tyrosine kinase) receptor (EGFR), which is greatly involved in cell transformation, differentiation and proliferation [145,146]. Therefore, this kind of S-PSs could be used as anti-tumor and anti-metastatic therapeutical/preventing agent, which might act either on tumour cells or by stimulating the immune response [147]. Further, the S-PSs from E. bicyclis and several other algae have demonstrated their potent bioactivity against different kinds of tumours, including lung and skin, both invitro and in-vivo [55,148-150] causing apoptosis in various tumour cell-lines [151,55,152].

The mechanisms involved in this antitumor activity might be associated again with the production of pro-inflammatory interleukins IL-2 and IL-12 and cytokine interferon-gamma (INF-γ) by the immune-stimulated macrophages, together with the increase of the activity of the natural killer cells (NK cells) and the induction of apoptosis [55,21]. NK cells can also upregulate the secretion of IFN-γ, which can activate either the T-cells for the production of IL-2 or the macrophages, which, after being activated, keep on producing IL-12 and activating NK cells [153,154]. The enhancement of the cytotoxicity of these NK cells (lymphocytes and macrophages) can be stimulated by other S-PSs such as fucoidans and carrageenans from other algae [129,137]. PSs can also activate some signaling receptors in the membranes of macrophages, such as Toll-like receptor-4 (TLR-4), cluster of differentiation 14 (CD14), competent receptor-3 (CR-3) and scavenging receptor (SR) [155]; these are also activated by other intracellular pathways, involving several other protein-kinases, that enhance the production of NO, which, in turn, plays an important role in causing tumour apoptosis [155]. These immunomodulation properties of S-fucoidans could be used for the protection of the damaged gastric mucosa as it was already demonstrated by using rat-models [156]. More information on the pathways and mechanisms responsible for the immune-inflammatory activities, including the involvement of the complementary system, may be found [60]. The anti-adhesive properties of some S-PSs, especially fucoidans might also explain their anti-metastatic activity, both in-vitro and in-vivo, in various animal models [157,33], as they can inhibit the adhesion of tumour cells to platelets, thus decreasing the possibilities of proliferation of neoplastic cells. The mechanisms by which fucoidans and other S-PSs exert their anti-adhesive ability were well documented by Li et al. [54]. Some researchers also highlighted the mitogenic properties and the cytotoxicity and tumoricidal activity of some arabinogalactans and fucoidans as well [129,158], either in different cell-lines or various animal models.
The anti-adhesive properties of algal S-PSs may also be relevant as these polymers can block the adhesion of tumour cells to the basal membrane, thus demonstrating to impair implantation of tumour cells and metastatic activity by binding to the extracellular matrix [159]. For example, the S-PSs from Cladosiphon were shown to prevent gastric cancer in-vivo, since it inhibited the adhesion of H. pylori to the stomach mucosa of gerbils [160]. Metastasis appearance could also be reduced in vivo by sulfated-laminaran, a (1---3): (1---6)-β-D-glucan, because this compound inhibited the activity of heparanase, an endo-β-D-glucuronidase involved in the degradation of the main PSs component in the basal membrane and the extracellular matrix. The expression of this enzyme is known to be associated with tumour metastasis [161]. These anti-tumor properties may also be found in some PSs from platensis, which are inhibitors of cell proliferation [78]. Other S-PSs, such as S-PSs p-KG03 from G. impudicum, has also anti-proliferative activity in cancer cell lines and inhibitory activity against tumour growth [128,162,163]. Other PSs from algae, such as C. vulgaris, and S-PS or LMW-derivatives of S-PS from P. cruentum, for example, are described as having similar properties [38]. In some research work, the immunomodulatory activity was associated to the ability of inhibiting carcinogenesis. Jiao et al. [47] found that a sulfated-rhamnan and some derivatives from the green seaweed E. intestinalis suppressed tumour cell growth in-vivo, but they did not show any toxicity against tumour cells in-vitro.

The oral administration of the S-PSs to mice enhanced the spleen and thymus indexes, and also induced the production of TNF-α and NO in macrophages, increased lymphocyte proliferation, and enhanced TNF-α release into serum. The degree of sulfation may play some role in the carcinogenesis process, although the action of the S-PSs may also depend on the type of tumour. In fact, an over S-PSs demonstrated the capacity of inhibiting the growth of L-1210 leukaemia tumour in mice, but, on the other hand, it was unable to inhibit the growth of Sarcoma-180 tumour in mice [149,123]. In addition to the sulfation level, MW may also influence the anticancer activity. For instance, LMW-PS derivatives showed to enhance anti-tumor activity [164]. On the other hand, the increment in the anticancer activity greatly depends on the conditions of the PSs depolymerisation [165]. Kaeffer et al. [82] suggested that the in-vitro anti-tumor activity of LMW-PS sulfated or not, against cancerous colonic epithelial cells might be associated with the inhibition of tumour cells proliferation and/or differentiation.
Lentinan can also increase engulfing ability of certain immune cells to search and destroy migratory cancer cells in the human body [166,167]. Treatment with lentinan can also enhance production of chemical messenger such as nitric oxide to stimulate the immune system [140,168]. In addition, the immune-activating ability of lentinan may be linked with its modulation of hormonal factors, which are known to play a role in cancer growth. The anticancer activity of lentinan is strongly reduced by administration of hormones such as thyroxin orhydrocortisone [169]. Moreover, lentinan can also enhance the immune response to the presence of cancer cells in the body by triggering cancer-specific reactions to fight against them. The mechanism of anti-cancer activity of lentinan is summarized in Figure (1) [170]. Overall, lentinan can suppress the growth and even kill cancer cells directly via multiple pathways involving activation of human immune system by different mechanisms such as stimulation of various immune cells and production of cell signal messengers [171].
Anticoagulant and antithrombotic activities
There are several studies on the anticoagulant properties of PSs isolated from algae, presented in a recent review [72] by different researchers: Cumashi et al. [33], Athukorala et al. [172], Costa et al., [68], Wijesekara et al., [42] and Wang et al. [41]. The main sources of the S-PSs from green algae with anticoagulant properties are Codium and Monostroma [143,144]. Some of the PSs, such as S-rhamnans, showed their action by extending the clotting time via the intrinsic and extrinsic pathways [174]. In fact, Codium spp present strong anticoagulant effects [175,176], but other species from Chlorophyta also contain S-PSs (native, LMW or otherwise modified) with anticoagulant properties. The mechanism of action of the referred PSs is mostly attributed to either a direct inhibition of thrombin or by enhancing the power of antithrombin III [177,178]. Some other PSs from green seaweeds also showed potent anticoagulant properties but their mechanisms of action are associated not only to a direct increase in the clotting time (APTT assays) by inhibiting the contact activation pathway, but also by inhibiting the heparin cofactor II-mediated action of thrombin [179,180] thus showing a potent antithrombotic bioactivity. In addition to their anticoagulant properties demonstrated in-vitro by APTT and TT tests, several S-PSs from algae of different groups present antithrombotic qualities in-vivo [181,50] by increasing the time of clot formation. In fact, Wang et al. [41] published an exhaustive work on this issue by including a summary table with 24 references about both the anticoagulant, and anti-and prothrombotic activities of several S-PS from various green algae.
In two other studies, Costa et al. [68] & Wijesekara et al. [42] also included the S-PS from brown and red algae that present effects on the blood clotting time. Wijesekara et al. [42] referred to the fact that there are few reports on the interference of PSs from algae on the PT (prothrombin) pathway, meaning that most of the marine S-PSs may not affect the extrinsic pathway of coagulation [42]. As a matter of fact, Costa et al. [68] did not detect any inhibition in the extrinsic coagulation pathway (PT test), for the concentrations used; only C. cupressoides increased the clotting time. Also, they found no anticoagulant properties (APTT and PT assays) in the S-PS from S. filipendula (brown algae) and G. caudate (red algae). Additional, in our laboratory we found no anticoagulant properties in the S-PSs from different strains of the red algae P. cruentum, despite the high content in sulfate and molecular weight. As Costa et al. [68] observed this could be due to the absence of sulfate groups in the monosaccharides at the non-reducing ends of the branches, which impaired the interaction between target proteases and coagulation factors. Nishino et al. [87] & Dobashi et al. [182] defended that there might be no effect above an upper limit for the content in sulfate, since the difference in the anticoagulant and antithrombotic activities decreased with the increase of the sulfate content. It seems that some of the chemical and structural features of the S-PSs may have some influence on their anticoagulant and/ or antithrombotic activities. The degree and distribution pattern of sulfate, the nature and distribution of monosaccharides, their glycosidic bonds and also the molecular weight showed to play some role on the coagulation and platelet aggregation processes induced by sulfated-galactans and sulfated-fucoidans [68,183,184]. In fact, at least for some fucoidans, the anticoagulant properties are related to the content in C-2 and C-2, 3 di-sulfates, this last feature being usually common in these PSs [185,186,131]. Several other studies documented the anticoagulant activity and inhibition of platelet aggregation [54,111,43], supplying more information on the mechanisms of different S-PSs for these biological activities. HMW-PS usually presents stronger anticoagulant activity [187] and if PSs has a more linear backbone, a longer polymer is required to accomplish the same anticoagulant effects [88]. On the other hand, both the native PSs and LMW-derivatives of M. latissimum presented strong anticoagulant activities [188]. Nishino and colleagues also observed that HMW fucans (27 and 58 kDa) showed greater anticoagulant activity than the ones with LMW (~10 kDa) [189].
They found that a higher content of fucose and sulfate groups coincided with higher anticoagulant activities of fractions from E. kurome [189]. However, despite its high sulfation level, the galactofucan from U. pinnatifida lacks significant anticoagulation activity [159]. In addition, a sulfated-galactofucan from schröederi did not present any anticoagulant properties in-vitro but demonstrated a strong antithrombotic activity when administered to an animal model during an experimental induced venous thrombosis, this effect disappearing with the desulfation of the polymer [190]. As for other PSs, the anticoagulant properties of the PSs from marine algae may not only depend on the percentage of sulfate residues, but rather on the distribution/position of sulfate groups and, probably, on the configuration of the polymer chains [72]. Spirulan from A. platensis is one of the PSs from microalgae that strongly interfere with the blood coagulation-fibrinolytic system and exhibits antithrombogenic properties [97], then, promising to be an anti-thrombotic agent in clots’ breakdown, although care should be taken regarding hemorrhagic strokes [38].
Figure (4) summarizes the preponderant target sites for the S-PSs from marine organisms on the coagulation system. Blue and red arrows indicate anticoagulant and pro-coagulant effects, respectively. (+) indicates activation and (−) indicates inhibitory effects. Anticoagulant effect: SG and FCS inhibit the intrinsic tenase and prothrombinase complexes [202,203]. It is still unclear if sulfated fucans (SF) have similar effects. These PS also potentiate the inhibitory effect of antithrombin (AT) and/or heparin cofactor II (HCII) on thrombin [199,204]. Their effects on factor Xa are very modest. The serpin-independent action preponderates on the plasma system. Pro-coagulant effect: SG and FCS activate factor XII [205,206]. This effect may result in severe hypotension (due to bradykinin release) and pro-coagulant (pro-thrombotic) action. It is unclear if SF activates factor XII. SF inhibits Tissue Factor Protease Inhibitor (TFPI), a specific inhibitor of the extrinsic tenase complex. So, SF has a pro-coagulant effect [207,208]. Of course, further studies are necessary to investigate whether this distinct mechanism of action may confer favorable effects to the PSs for the prevention and treatment of thromboembolic events. In particular, it is necessary to clarify which one of the two mechanisms (serpindependent or serpin-independent) is more favorable for an antithrombotic therapy.
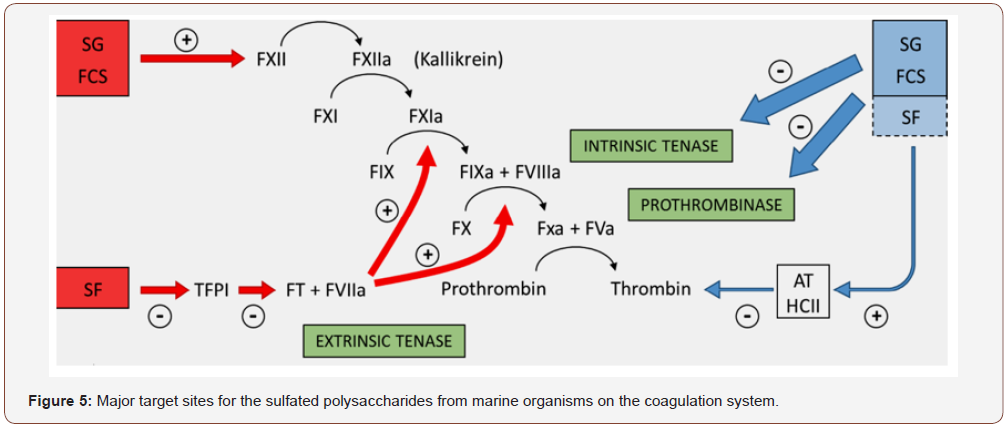
Antilipidaemic, hypoglycemic and hypotensive activities
S-PSs from algae are potent inhibitors of human pancreatic cholesterol esterase, an enzyme that promotes its absorption at the intestinal level; this inhibitory effect is enhanced by higher molecular weights and degree of sulfation [2]. A sulfated-ulvan from U. pertusa in an in-vivo study using mice models regulated the ratio HDL/LDL-cholesterol and reduced the levels of triglycerides (TG) in serum [209]. On the other hand, in another experiment with rats and mice, using native ulvans from the same species, the animals experienced a hypocholesterolaemic effect but no reduction in the TG profile [210]. An opposite reaction was observed when the PSs was acetylated and over sulfated, as TG levels were normalized. It seems that the ability to sequester bile extracts may be involved [210]. The contents in sulfate and acetylate groups play important roles during the dislipidaemia process [211,212]. Ulvans from Ulva spp also showed antiperoxidative properties, preventing liver tissues from hyperlipidaemia, including that induced by toxic chemicals and protecting the injured tissue from the oxidative stress [213], and improving antioxidant performance of the animal models. In fact, these S-PSs regulated superoxide dismutase (SOD) and catalase, increased vitamins E and C, and reduced glutathione, and had some role in reducing the levels of aspartate and alanine transaminases in the rats’ liver [214,209]. Additionally, the S-PSs from M. nitidum also demonstrated hepatoprotective activity by increasing the expression of liver detoxifying enzymes, and, therefore, showed to be good agents for chemoprevention medicine [215].
The activity of these PSs may be related to their uronic acid and sulfate content, which are able to sequester and bind to bile acids [216], reducing their levels. Other S-PSs from green algae also revealed hypolipidaemic properties, such as that from E. prolifera. These PSs regulated the lipidic profile both in plasma and liver, increasing HDL-cholesterol, in rats [217]. Fucoidans from L. japonica, the native or LMW-derivate, have hypolipidaemic effects, decreasing total and LDL-cholesterol in the serum and TG in rats [218], and they prevented hyperchole-sterolaemia in mice [133]. Another mechanism to reduce blood cholesterol in humans by S-PSs is associated to their high capacity to inhibit pancreatic cholesterol esterase, which is responsible for the absorption of cholesterol and fatty acids at the intestine [2]. It seems that the presence of sulfate at the C-3 position of the sugar residues greatly enhances that inhibition [2]. Porphyran from P. yezoensis has antihyperlipidaemic properties [219,220] by reducing the release of apolipoprotein-B100 (apoB100) and decreasing the synthesis of lipids in human liver cultured cells [221]. By reducing the secretion of apoB100, porphyran has the potential to be used as a therapeutic agent to treat CVD. Additionally, some types of carrageenans have already proved to decrease blood cholesterol in humans [222] and in rats fed on a diet enriched with a mixture of κ/λ- carrageenans from G. radula [223]. Most of the PSs from marine algae are naturally highly sulfated, with high molecular weights, making them not easily absorbable and thus enabling them to be used as anticholesterolaemic agents. Few studies were carried out in this area, namely focusing on Porphyridium, P. cruentum, R. reticulata [224-227], but these suggest a strong potential of S-PSs from unicellular algae to be used as hypolipidaemic and hypoglycaemic agents, and as promising agents for reducing coronary heart disease, due to their hypocholesterolaemic effects [72]. As far as we know, scarce research was performed on the mechanisms underlying the antihyper-lipidaemic activity. However, the sequestration and disruption of the enterophatic circulation of the bile acids may be involved [209,228,229]. Ulvans and their LMW-derivatives, and also the S-PSs from Porphyridium showed to increase the excretion of bile [230,210]. Another explanation for the antihyperlipidaemic activity of S-PSs may be associated to the fact that they can effectively increase the anionic charges on the cell surface, which improve the removal of cholesterol excess from the blood, thus, resulting in a decrease of serum cholesterol [54]. In addition, most PSs have ion exchange capacity, such as those from Porphyridium and Rhodella [231], and they can function as dietary fibres. This could also explain the ability to lower down cholesterol [232]. PSs may act as dietary fibres, immunostimulating the goblet cells in the intestine to increase the release and effects of mucin [233]. Moreover, the administration of PSs may increase the viscosity of the intestinal contents, interfering with the formation of micelles and nutrient absorption, thus, lowering lipid absorption, and reducing gastrointestinal transit time (GTT) [230,57]. Other PSs have the ability to inhibit the enzyme α-glucosidase, thus improving the postprandial hyperglycaemia [234], and another can also reduce the blood pressure by inhibiting the release of plasma angiotensin II [235].
Wound healing and wound dressing
Due to their inherent biocompatibility, low toxicity, and pharmaceutical biomedical activity, various PSs, suchas chitin, chitosan, cellulose, hyaluronan, and alginate, have been widely used to prepare wound healing materials [236,142,237]. Hyaluronan, a major extracellular component with unique hygroscopic, rheological, and viscoelastic properties, has been extensively developed for tissue repair purposes due to its physicochemical properties and specific interactions with cells and extracellular matrix. It is generally accepted that hyaluronan plays multifaceted roles in the mediation of the tissue repair process and is involved in all the stages of wound healing, i.e. inflammation, granulation tissue formation, reepithelialization, and remodeling. Derivatives of hyaluronan, such as cross-linked, esterified or other chemically modified products have also been developed for tissue repair or wound healing purposes [238,239]. Remarkably, wound healing promoting activity of the materials is also important in the designing of materials for tissue engineering. All-natural composite wound dressing films prepared by dispersion and encapsulation of essential oils in sodium alginate matrices have been reported to show remarkable antimicrobial and antifungal properties and may find applications disposable wound dressings [240].
Chitosan/silk fibroin blending membranes crosslinked with dialdehyde alginate have been developed for wound dressing and the membranes were found to promote the cell attachment and proliferation, which suggests a promising candidate for wound healing applications [241]. Blending aqueous dispersions of sodium alginate and povidone iodine (PVPI) complex was prepared as free standing NaAlg films oras Ca2+ cross-linked alginate beads. These products were demonstrated to show antibacterial and antifungal activity and controlled release of PVP Iinto open wounds when the composite films and beads were brought into direct contact with water or with moist media [240]. This proved that they could be suitable for therapeutic applications such as wound dressings. In situ injectable nano-composite hydrogels composed of curcumin, N, O-carboxymethyl chitosan, and oxidized alginate as a novel wound dressing was successfully developed for dermal wound repair application [139]. In-vitro release, in-vivo wound healing, and histological studies all suggested that the developed nanocurcumin/ N, O-carboxymethyl chitosan/ oxidized alginate hydrogel as apromising wound dressing might have a potential application in the wound healing. Silver nanoparticles containing polyvinyl pyrrolidone and alginate hydrogels were synthesized using gamma radiation and showed the ability of preventing fluid accumulation in exudating wound [242]. The incorporation of nanosilver particles provided as trong antimicrobial effect and therefore made such polyvinyl pyrrolidone/alginate hydrogels suitable for use as wound dressing. Except the alginate and its various derivatives, other natural PSs such as cellulose, chitin, chitosan, and hyaluronic acid have also been explored for wound dressingor wound healing applications [243-245].
Antioxidant activity
Oxidation is an essential process for all living organisms for the production of energy necessary for biological processes [246]. In addition, oxygen-centered free radicals are involved in development of a variety of diseases, including cellular aging, mutagenesis, carcinogenesis, coronary heart disease, diabetes and neurodegeneration [247]. Though almost all organisms possess antioxidant defense and repair systems to protect against oxidative damage, these systems are often insufficient to prevent the damage entirely [98]. Recently, much attention was paid to screening natural biomaterials in the case of several clinical situations since use of synthetic antioxidants is restricted due to their carcinogenicity [135]. Among various natural antioxidants, PSs in general has strong antioxidant activities and can be explored as novel potential antioxidants [248,249]. Recently, PSs isolated from fungal, bacterial and plant sources were found to exhibit antioxidant activity and were proposed as useful therapeutic agents [250,251].
essential process for all living organisms for the production of energy necessary for biological processes [246]. In addition, oxygen-centered free radicals are involved in development of a variety of diseases, including cellular aging, mutagenesis, carcinogenesis, coronary heart disease, diabetes and neurodegeneration [247]. Though almost all organisms possess antioxidant defense and repair systems to protect against oxidative damage, these systems are often insufficient to prevent the damage entirely [98]. Recently, much attention was paid to screening natural biomaterials in the case of several clinical situations since use of synthetic antioxidants is restricted due to their carcinogenicity [135]. Among various natural antioxidants, PSs in general has strong antioxidant activities and can be explored as novel potential antioxidants [248,249]. Recently, PSs isolated from fungal, bacterial and plant sources were found to exhibit antioxidant activity and were proposed as useful therapeutic agents [250,251]. The main mechanism by which S-PSs from green algae exert their primary antioxidant action is by scavenging free-radicals (DPPH-radicals) or by inhibiting their appearance [251]. They also demonstrated to have total antioxidant capacity, and a strong ability as reducing agents and as ferrous chelators [251]. However, some S-PSs, such as sulfated-heterogalactan from C. cupressoides do not show a good scavenging power, but they are rather powerful against reactive oxygen species (ROS) [252]. It is interesting to note that fucoidans from brown algae seem to exert a reducing power bigger than the S-PSs from other groups [68]; the PSs from S. filipendula has an effect even stronger than vitamin C. Moreover, the fucoidan from L. japonica has a great potential to be used in medicine in order to prevent free-radical mediated diseases, as it successfully prevented peroxidation of lipids in plasma, liver and spleen in-vivo, despite showing no effects in-vitro
The S-PSs from S. fulvellum has shown a NO scavenging activity higher than some commercial antioxidants [253]. In addition, the S-PSs from the red algae P. haitanensishas demonstrated to decrease antioxidant damages in aging mice [254]. It seems that LMWS- PS may present higher antioxidant activity than the native polymers, as it was verified with the PSs from U. pertusa and E. prolifera [255,256]. It is probably related with the ability of PSs to be incorporated in the cells and to donate protons [42]. As noted by Raposo et al. [72], S-PSs produced and secreted out by marine algae have shown the capacity to prevent the accumulation and the activity of free radicals and reactive chemical species. Hence, S-PSs might act as protecting systems against these oxidative and radical stress agents. The S-PSs from Porphyridium and Rhodella reticulata exhibited antioxidant activity [257,258], although some research revealed no scavenging activity and no ability to inhibit the oxidative damage in cells and tissues for the crude S-PSs with high molecular weight from P. cruentum, while the PS-derived products after microwave treatment showed antioxidant activity [259].
In all cases, the antioxidant activity was dose dependent. PSs from A. platensis also exhibit a very high antioxidant capacity [260]. Due to their strong antioxidant properties, most of the S-PSs from marine algae are promising since they may protect human health from injuries induced by ROS, which can result in cancer, diabetes, some inflammatory and neurodegenerative diseases, and some other aging-related disorders, such as Alzheimer and CVD. The influence of sulfate content on the antioxidant activity depends rather on the origin of the PSs. For example, the PS from U. fasciata and other algae with lower sulfate content demonstrated a strong antioxidative power [261,262,258,259], while the antioxidant activity observed in PSs from E. linza and other seaweeds showed to be sulfate-dependent [263,264]. Furthermore, high sulfated PSs were shown to have an enhanced scavenging power [251,265], this property being also dependent on the sulfate distribution pattern [68]. It seems, in addition, that the protein moiety of PSs may play some role on the antioxidative power. For example, Tannin-Spitz et al. [258] reported a stronger antioxidant activity for the crude PSs of Porphyridium than for the denatured PSs. Zhao et al. [266] found that the antioxidant activity of S-PSs was apparently related, not only to MW and sulfated ester content, but also to glucuronic acid and fructose content. This antioxidant activity seems to be attributable to metal chelating, free radical and hydroxyl radical scavenging activities of the S-PSs.
Toxicity of polysaccharides
The toxicity of polysaccharide is very crucial to the development of any product for the medical treatments. An animal experiment was conducted to evaluate the toxicity of polysaccharide and the results found that no toxicity was exhibited to the liver, kidney, heart, thymus or spleen of the mice which were fed with the polysaccharide conjugate and none of the mice died throughout the period of the experiment. There was no significant difference between the thymus index, spleen index and liver index of the mice from the test and control groups. It might be a candidate of dietary supplements besides the bioactivities as a polysaccharide [267- 293].
To read more about this article...Open access Journal of of Agriculture and Soil Science
Please follow the URL to access more information about this article
https://irispublishers.com/wjass/fulltext/new-trends-of-the-polysaccharides-as-a-drug.ID.000572.php
To know more about our Journals...Iris Publishers
To read more about this article...Open access Journal of of Agriculture and Soil Science
Please follow the URL to access more information about this article
https://irispublishers.com/wjass/fulltext/new-trends-of-the-polysaccharides-as-a-drug.ID.000572.php
To know about Open Access Publishers
No comments:
Post a Comment