Authored by Ruhul A Khan*,
Abstract
Over the last century and a half, human have learned how to make synthetic polymers into plastic products. Synthetic polymer and plastic products are resistant to degradation and causes the environmental pollution. As growing awareness about ecological and environmental issues spread, the persistence of plastic waste began to trouble observers. Researchers and innovators are searching for a way to place more environmentally friendly biodegradable materials than conventional plastics in the market. Following that, biodegradable biocomposites have entered the market for use in agriculture, medicine, packaging, civil engineering, and other areas. Recently, natural fiber or, lignocellulosic fiber-based biocomposites have gained much attention for different industrial uses and applications because of their low density and renewability and significant advantages of natural fibers. These fiber-reinforced biocomposites are having satisfactory physical and mechanical properties (i.e., tensile properties, flexural stress-strain behavior, fracture toughness, fracture strength, impact strength) which make them more sustainable and attractive than other composites with a remarkable property of its degradability in the environment. This review is intended to provide a brief discussion of biocomposites, natural fiberreinforced biocomposites, lignocellulosic fiber-based biocomposites, various fabrication processes of fiber-reinforced biocomposites, significant physical and mechanical properties of fiber-reinforced biocomposites, materials used for biocomposites, and its future development.
Keywords: Composites; Fiber reinforced biocomposites; Biocomposites; Biodegradable materials; Manufacturing techniques; Material selection; Future development
Introduction
Mankind has always searched and investigated to get materials for uses at low prices with better quality but sometimes this causes environmental hazardous. For the augmentative use of petroleumbased products, depletion of petroleum resources and entrapment of plastics in the environment rising at an alarming rate. Pollution problems that are increasing day by day responsible for the use of plastics and the burning of fossil fuels are affecting our day-today life and threatening human rights to live. Availability of raw materials, cheap rate, and easy to use of plastic products, though every class of people can use it an increased amount of plastic waste that causes a negative impact on Bangladesh and also affects Global environment. European Union as a whole and many European governments, in particular, are recently changing conventional policies and revising the policy options to minimize the plastic waste problems [1]. Scientists and researchers are always working for decreasing the use of conventional plastic products and invented various alternative ways that already took more space for plastics and still now discovering advanced methods that will help to replace plastics in the near future [1].
Composite materials are becoming popular for their unique characteristics than conventional plastics and in these materials, a homogeneous matrix is reinforced by a stiffer and stronger constituent which is usually fibrous in nature. For instance, Fiberreinforced plastics (FRPs) or fiber-reinforced polymer are the materials formed by incorporating fibers like glass, carbon, wood, etc. in a polymer matrix based on epoxy, vinyl, etc. FRPs are replacing conventional stainless steel, premium alloys, carbon steels, etc. [2].
Replacing conventional plastics with biodegradable materials is very challenging whereas the design of materials exhibits structural and functional stability during storage and use and these biodegradable materials are efficient to microbial and environmental degradation only upon disposal and with environment-friendly aspects. Naturally, these composite materials can’t be used as fibers alone, typically fiber is impregnated by a matrix material that works to transfer the load to the fibers. Matrix also restrains the fibers from abrasion and environmental impact. Glass and metal are used as matrix materials, but for these high prices and restriction of use to R&D laboratories, polymers are much more commonly used having the majority of low-to-medium performance applications and epoxy or more sophisticated thermosets having the higher demand in the market. During recent years several significant efforts have been made to place some of the biodegradable composites using renewable resources instead of synthetic composites. Fiber acts as reinforcement by giving strength and stiffness to the material structure while the plastic matrix serves as the adhesive to hold the fibers in place so that suitable structural components can be made in the fiber-reinforced plastic composites. In respect of balancing the cost and acquire suitable properties for different purposes and applications, biocomposites are the possible solutions. Biocomposites are defined as a special class of composite materials that are obtained by blending natural fibers or, biofibers or, lignocellulosic fibers with bio-based polymers. These materials represent an ecological, environment-friendly, and low-cost alternative to conventional petroleum-derived materials. For these reasons, the end uses of these materials are progressively increasing. These several articles show some reviews of various natural fiber-reinforced biocomposites [3-8].
The biocomposites which are produced using a reinforcement from renewable resources and a biopolymer matrix over the reinforcement are now an extensive research and development area due to the promising mechanical properties, recyclability after service, biocompatibility, and biodegradability of these materials. Various potential applications of these composites in different fields such as automotive, packaging, and household goods. Natural fiber-reinforced biocomposites can be used as low-cost materials with different structural properties at the same time. In this paper, a review of biocomposites and natural fiber-based reinforced biocomposites is importantly studied. This paper emphasizes the composites, biocomposites, natural fiber-based reinforced biocomposites, lignocellulosic fiber-reinforced biocomposites, various fabrication processes of fiber-reinforced bio composites, physico-mechanical properties of fiber-reinforced biocomposites, materials selection for biocomposites and also addresses future developments of fiber-reinforced biocomposites [9-12].
Composites
Composite material has primarily consisted of two distinct materials, a fiber which acts as reinforcement, and a matrix that behaves like a binder each with its mechanical properties such as stiffness and strength. When a reinforcement and a matrix are combined to make a composite, then the mechanical properties not just only depend on those of the two materials being mixed or, blended, but on the relative amount of each material, the size and shape of the reinforcement, and its orientation concerning the loads that are to be applied to the composite. Fibers; natural or, synthetic, provide strength and stiffness and act as reinforcement in fiberreinforced composite materials; ultimately composite material properties are governed by the inherent properties of these fibers. The matrix material can be defined as a continuous phase that includes polymer matrix composites materials, metal matrix composite materials, and inorganic non-metallic matrix composite by the different matrix materials. A composite has a polymeric, metallic, and ceramic matrix. In short, composite material is a multi-phase system consisted of reinforcing material and matrix material. In most cases, the reinforcement is harder, stronger, and stiffer than the matrix. The mechanical properties of composite materials are a major function of the shape and dimensions of the reinforcements [13-16].
Natural fibers such as wood and some synthetic composites have been used by humans for thousands of years, but major developments have been taken place in recent times. All vegetable fibers, whether from wood or non-wood origin, are composed of three main cell wall polymers: cellulose, lignin, and matrix polysaccharides (such as pectins and hemicelluloses) which are associated with cellulose and lignin in the cell wall. In addition, a number of non-structural components, such as waxes, nitrogenous substances, and inorganic salts, as extractives, are also present. In fiber structure, vegetable fibers are viewed as miniature composites composed of millions of fibrous units known as microfibrils [17- 21]. The required values of the stiffness and tensile strength of a composite are the selection parameters of suitable fibers. Elongation at failure, adhesion of fibers and matrix, thermal stability, dynamic and long-term behavior, price, and processing costs are the further criteria of the choice of a suitable reinforcing fiber for composite material. When it comes to comparing the tensile strength, elasticity, and elongation at break of natural fiber with synthetic fibers, becomes very clear that hemp and flax fibers can potentially compete with E-glass fibers, which show as a reference because of their important roles in composite technology [22].
Industrial composite materials are mainly based on thermosets, thermoplastics, and polymeric matrices. These materials are usually reinforced with aligned ceramic fibers, such as carbon or, glass. Commonly exhibit marked anisotropy, since the matrix is much weaker and less stiff than the fibers. Interest in metal matrix composites (MMCs) has been increasing in recent years, such as aluminum reinforced with short fibers or, ceramic particle and titanium-containing long, large-diameter fibers. The important features of composites are markedly different from those of the constituents and are determined by the properties of the constituents, the microstructure (which includes morphology of the reinforcement and the volume fraction), and the matrix reinforcement interface properties [23,24].
Biocomposites
This century has witnessed remarkable improvements due to the concerns about environmental and sustainability issues in green materials in the field of polymer science through the development of biocomposites. Major and significant advantages of these biocomposites can be easily disposed of or composted at the end of their lifecycle without harming the environment that is not possible with synthetic fiber-based polymer composites. The statistical data of Municipal solid waste (MSW) landfills represent the dominant option for waste disposal in many parts of the countries, 70% of MSW in Australia has been shown to landfills without pretreatment in 2002 [7,25-26].
Biocomposites are naturally fully degradable composites that are made up of natural fibers as reinforcing phases with a biopolymer matrix. A material is defined as a biocomposite composed of two or more distinct constituents (one being naturally derived) which are combined to yield a new material with improved performance over individual constituent materials. The constituent materials are the matrix and reinforcing components. The primary load-carrying element the reinforcing component, which can be in the form of fibers, particles, whiskers, and flakes. The matrix serves to bind the reinforcing components together and provide mechanical support [27,28].
These biocomposite materials have not only been produced by combining the natural fibers and polymers but also there are several examples where two natural polymers have been combined to make a biocomposite with improved mechanical and gas barrier properties. Rice proteins, wheat gluten, egg albumin have been combined with starch to improve the functional properties of the composite. An hourly studied biocomposite is a natural-fiberreinforced biopolymer composite. This reinforcing component is a natural fiber or cellulose extracts combined with a bioplastic matrix. As natural fiber has good tensile strength, elongation% adds further strength to the weaker biopolymer matrix and allowing for the biocomposite material to be used in more applications [29-34].
In recent times, researchers and scientists focus on embedding nanoparticles or particulates such as layered silicates, hydroxyapatite, carbon nanotubes, cellulose, and talc into bioplastics. PLA bioplastics (most commonly used) is layered silicate clay, as it has been attributed to a dramatic increase in material properties such as improved tensile and flexural properties, enhanced barrier properties, elevated heat distortion temperature, and accelerated biodegradation. These nano-biocomposites are distributed and dispersed of the reinforcement within the biopolymer [35].
Fiber reinforced biocomposites
Fiber-reinforced biocomposites contain reinforcements having lengths higher than cross-sectional dimensions. Fibrous reinforcement represents physical rather than a chemical means of changing material to suit various engineering applications [36].
Natural fiber reinforced biocomposites
Natural fibers such as jute, kenaf, hemp, oil palm, pineapple, flax, sugarcane, and leaf, etc. are cheap, environmentally friendly, renewable, partially and completely biodegradable which can be utilized to obtain new high-performance polymer materials. The significant characterizations of natural fiber composite materials are eco-friendly, lightweight, strong, renewable, cheap, biodegradable, and sustainable. It has comparatively better properties compared to synthetic fiber. Recently, many researchers and scientists are using natural fibers as an alternative reinforcement in polymer composites due to their advantages over conventional synthetic materials. These natural fibers include jute, hemp, banana, bamboo, sisal, kenaf, coir, flax, sugarcane and many others which provide good mechanical properties compared to man-made fibers and their cost are effective and reasonable, recyclable and renewable, reduce energy consumption, less health risk, non-irritation to the skin and nonabrasive to the equipment [8,36-38].
Natural fibers can be used as a reinforced material because of their thermoplastic and thermosetting behavior. Thermosetting resins such as epoxy, polyester, unsaturated polyester resin, polyurethane, and phenolic are commonly used for manufacturing composites material which gives a higher performance in various applications. They provide adequate mechanical properties and the price of these items is reasonable. Academicians, researchers, students, and also the Research and Development (R&D) section of industries are giving more attention to natural fibers-based composites over conventional synthetic fibers-based composites because of their good mechanical properties such as high strength, low density, and ecological advantages. Due to their bio-degradable, non-carcinogenic and use of natural composites is increasing day by day. In 2010, U$2.1 billion natural fiber reinforced polymer composites (NFPC) used in the different industrial sector around the whole world, and after that industry keeps huge interest in the NFPC and after 5 years (2011-2016), the NFPCs industry has increased the use of natural fiber around 10% [39].
As its cost-effectiveness behavior, can be used in different sectors such as automobile, building, aerospace, packaging, and construction sectors, storage device, railway coach interiors and also is used as a replacement of high-cost glass fiber. Though natural fiber has several advantages (i.e. low cost, low density, biodegradability, etc.), has also some disadvantages as well such as high moisture absorption. Therefore, chemical treatments are essential to control high moisture absorption. The mechanical properties of the natural fibers are greatly affected by many factors such as fiber length, fiber-matrix adhesion, fiber aspect ratio, etc. after chemical treatment. Several research works have been already done using natural fiber. Furthermore, using these fibers can be formed into light composites leading to weight reductions and, especially in the automotive field, fuel-saving [40-42].
Effective benefits of being biodegradable and produced from renewable resources, natural fibers are also less abrasive to processing equipment than synthetic fibers like glass or ceramic fibers and traditional fillers like mica and glass, and they exhibit a lower density, which makes biocomposites lightweight, economical materials and environmentally friendly [43].
Natural fibers used for biocomposites
As natural fibers are found from plant, animal, and mineral sources, so according to their resources natural fibers can be divided and classified into three main classes. They are plant fibers, animal fibers, and mineral fibers. Among natural fibers lignocellulosic natural fibers are widely used as reinforcements for making biocomposites materials [44].
Lignocellulosic natural fibers: Lignocellulosic fibers (LCFs) are those fibers that indicate the predominance of cellulose and lignin in natural plant fibers and these fibers can reach tensile strength values near close to the glass fiber [3].
Among natural fibers, lignocellulosic fibers are investigated as promising substitutes of the synthetic fibers in polymeric composites and several studies have examined the drawbacks and advantages of the most significant lignocellulosic fibers and their related polymeric composites. As mentioned before the main three classes of natural fibers and it’s known that all types of plant fibers are composed of cellulose and animal fibers contain protein. Lignocellulosic fibers have three primary chemical constituents, namely cellulose (α-cellulose), hemicelluloses, and lignin. Besides, lignocellulosic fibers also contain a minor amount of pectin, waxes, and water-soluble substances. Bledzki & Gassan [3] indicated that a single fiber of a plant is a complex natural composite consisting of several cells. These cells are composed of cellulose microfibrils connected by lignin and hemicelluloses. The lignin is an amorphous binder with both aliphatic and aromatic polymeric constituents, whereas the hemicellulose comprises polysaccharides that remain associated with the cellulose even after lignin is removed. The ratio between cellulose and lignin/hemicelluloses as well as the spiral angle of the microfibrils vary from one natural fiber to another. Since these structural parameters determine the mechanical properties, each kind of LCF presents distinct properties [3,4, 44-46].
Lignocellulose fibers’ compositions and structures vary greatly, depending on plant species, age, climate, and soil conditions. The information on the chemical composition of lignocellulosic fillers and fibers is important because it determines their properties and thus their applications [12]. Table 1 lists the chemical composition of several lignocellulosic fibers.
Table 1: Chemical composition and structural parameters of common lignocellulosic fiber [7-8, 47-51].
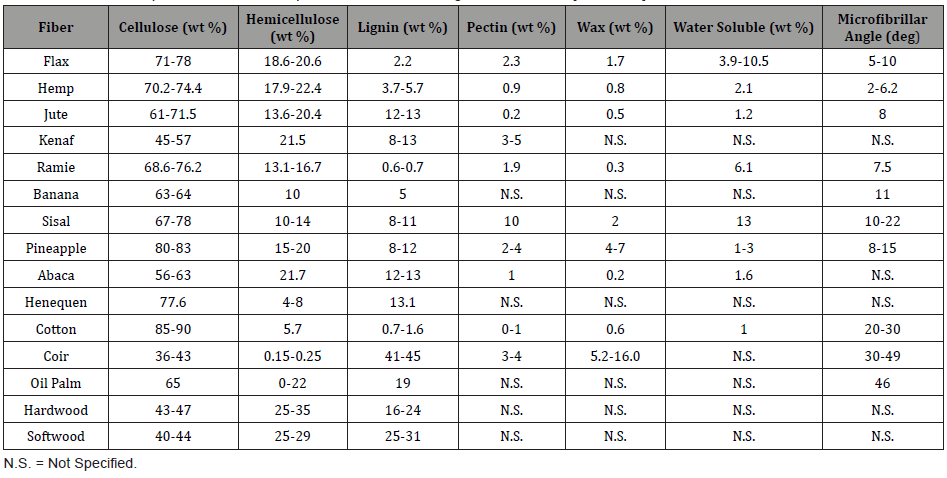
Fabrication of Fiber Reinforced Biocomposites
Various fabrication methods have been investigated for biocomposites. These are classified into two categories according to the types of reinforcement used: (i) particle or short fibers and (ii) continuous fibers. Woven fabric is formed from natural fibers that have been introduced as the reinforcements for the continuous fiber-reinforced bio-composites [52].
The fabrication process of laminated composite with four layers of jute woven fabrics has been introduced and done. By saturating in the resin matrix, the jute fabrics were treated with alkali in the biaxial tensile stress state. Therefore, the mechanical stiffness of the composite with the fibers treated with alkali has been improved under applied stress. From research results, it was found that leaf fiber (Pineapple leaf fiber or PALF, Henequen) based biocomposites show very high impact strength while bastfiber (Kenaf, Hemp, etc.) based bio-composites exhibit superior flexural and tensile properties. Proper pre-treatment of bio-fibers like alkali treatment (AT) and/or silane treatment (ST), the water absorption of the resulting bio-composites could be reduced [53,54].
For fabricating continuous or discontinuous fiber-reinforced composites, a commingled technique was developed for the preparation of thermoplastic composites such as PP. To prepare the continuous fiber composites, both the reinforcement and matrix fibers are mingled into yarns or fabrics, while both fibers are entangled into nonwoven mats for the preparation of chopped fiber composites. Heat and pressure are applied to the commingled preforms only the resin fibers within them melt and flow, forming a continuous matrix phase between the reinforced fibers for converting them into solid composites. This fabrication method is exploited to manufacture bio-composite material using a carding process, which is used to make uniform blends of discontinuous natural fibers such as jute or kenaf with synthetic fibers for use as the matrix (Figure 1) [55].

The commingled fabrication method using the carding process may be an effective means of processing bio-composites using long and discontinuous natural fibers because it can avoid the process of converting them into continuous yarns, which allows the cost to be reduced and the fibers to be uniformly distributed in the composites. Here long fibers refer to fibers whose length is large compared to short fibers with a length of a few millimeters at most [55].
The techniques used to manufacture biocomposites are based largely on existing techniques for processing plastics or composite materials. The manufacturing of natural fiber-reinforced biocomposites is based, in general, on the technologies used for conventional synthetic fiber composites. There are many processes to fabricate fiber reinforced biocomposite materials and these processes can primarily be classified into three main classes; Conventional Manufacturing Techniques, Advance Manufacturing Techniques, Automated Manufacturing Techniques. Typical processing techniques include Hand Lay-up, Spray-up, Pultrusion, Compression molding, Injection molding, Vacuum bug molding, Vacuum infusion molding, Resin transfer molding, Hot compression technique, sheet molding compounding, Solvent casting method, Electrospinning process, Additive manufacturing, Filament winding process [56].
Typical processing techniques include extrusion, injection molding, compression molding, pultrusion and filament winding, direct long-fiber thermoplastic (D-LFT) is suitable and investigated for natural fiber reinforced thermoplastic biocomposites [8,57]. On the other side, resin transfer molding (RTM) and sheet molding compound (SMC) is implemented with thermoset matrices. Processing methods and suitable processing conditions have a significant influence on the parameters (moderate temperatures (below 200°C), dispersion, orientation, and aspect ratio) that determine the mechanical properties of natural fiber-reinforced biocomposites [58].
Therefore, to choose a suitable process to fabricate natural fiber-reinforced biocomposites, design, and manufacturing engineers would mainly focus on several criteria including desired properties, size, and shape of resultant composites, the production rate, processing qualities of raw materials, and the manufacturing cost [59] (Table 2).
Table 2: Various fabrication techniques of Lignocellulosic fibers.

Conclusion
Plastics debris originates from both land and ocean which is usually found in marine environment (e.g. water bodies, beach sediment). It is very difficult to biodegrade the debris resulting persist in the environment. These debris bring negative impacts on the marine biota and their food web through plastic ingestion or entanglement. There are some marine organisms followed by fish, sea birds, or sea lions have settled ingested plastic that is often associated with hydrophobic contaminants in their bodies. Moreover, these organisms may also become entangled in the plastic which can be dangerous. At last, it is found that governments introduce laws to control the sources of plastic debris and the use of plastic debris. In parallel, plastics industries should take responsibility for the end-of-life of their products by introducing plastic recycling or upgrading programs.
Conventional manufacturing techniques
Conventional processing techniques are used for making composite materials, Fiber-reinforced plastics (FRP), Natural fiber reinforced composites (NFRC) and biocomposites include Hand Lay-up, Spray-up, Pultrusion, Compression molding, Injection molding, Vacuum bug molding, Vacuum infusion molding, Resin transfer molding, Hot compression technique, sheet molding compounding, Solvent casting method [56].
Hand lay-up: The simplest and oldest open molding method of the composite fabrication process is the hand lay-up method. By this process (Figure 2), low-volume, labor-intensive method suited especially for large components, such as boat hulls. Fiber or other reinforcing mat or woven fabric or roving is positioned manually in the open mold, and resin is poured, brushed, or sprayed over and into the chopped fiber where the secondary spray-up layer embedded the core between the laminates, resulting in the composite. To complete the laminate structure the entrapped air is removed manually with squeegees or rollers. Room temperature curing polyesters and epoxies are the most commonly used matrix resins. Curing is a process which is initiated by a catalyst in the resin system that hardens the fiber-reinforced resin composite without external heat. A pigmented gel coat is first applied to the mold surface for a high-quality part surface.

• A mould release agent is applied onto the mould surface for ease of removing of finished composite products.
• The laying-up of a component begins with applying gel coat to the mould surface.
• It is resin-rich layer and the purpose is to prevent the fibers appearing on the mould surface.
• The composites are then prepared by placing a fiber reinforcement and by adding polymer resin onto it.
• A roller is used to consolidate the composites and the composites are made layer by layer
. • The process is repeated with more layers until the desired thickness is obtained.
• Then the composites are cured at room temperature or in an oven.
• Thermosetting resins like unsaturated polyester, vinyl ester, and epoxy are among the most commonly used resins [67].
Spray-up process: The spray-up technique is a similar process to hand lay-up. A handgun is used that sprays resin and chopped fibers on a mold. Simultaneously, a roller is also used to fuse these fibers into the matrix material. The process is illustrated in Figure 2. This technique is an open mold type of technique, where chopped fibers provide good conformability and quite faster than the hand lay-up method [56,68].
Pultrusion: Pultrusion is a combination of two words: pull and extrusion. It is a manufacturing process for producing continuous lengths of reinforced polymer structural shapes with constant cross-sections. Raw materials are a liquid resin mixture (containing resin, fillers, and specialized additives) and flexible textile reinforcing fibers. The process involves pulling these raw materials (rather than pushing, as is the case in extrusion) through a heated steel forming die using a continuous pulling device. While pultrusion machine design varies with part geometry, the basic pultrusion process concept is described in the schematic shown below (Figure 3):

• The process is similar to extrusion process, but in pultrusion, the product is developed by pulling the materials rather than pushing the materials through the die as in the case of extrusion.
• Continuous fiber rovings or tapes are pulled by means of a puller through a resin bath of thermosetting polymer.
• Examples of thermosetting polymers that can be used in this process include epoxy and unsaturated polyester.
• The resulting impregnated fiber composites leave the resin bath and are being pulled and passed through a series of forming dies.
• The shape of the final product follows the shape of the cross of the dies and the shape can be circular, rectangular, square, and I-shaped and H-shaped sections.
• The composite is also cured in one of the dies. The end products are normally in the form of rods and bars. At the end of the pultrusion process, the products are cut to the required lengths [56].
Compression molding: Compression molding is a common process used for both thermoplastic and thermoset stock shape materials.
• Compression molding is accomplished by placing the plastic material (can be a granular or pelletized form) in a mold cavity to be formed by heat and pressure. The process is someone similar to making waffles.
• The heat and pressure force the materials into all areas of the mold. The heat and pressure cycle of the process will harden the material and then it can be removed.
• Typically, thermosetting compounds like polyesters, phenolics, melamine and other resin systems are compression molded using alternating layers of different reinforcement materials to create a final product.
• However, there are various thermoplastics that are commercially compression molded as well.
• Compression molding offers a high degree of automation, short cycle times, good reproducibility and excellent dimensional stability for both thermoplastics and thermoset which is the reason for diverse applications in various industrial sectors including the automotive industry [69] (Figure 4).

Injection molding: Injection molding is a manufacturing process commonly used for fabricating items from plastic trinkets and toys to automotive body parts, cell phone cases, water bottles, and containers. Injection molding can also be used to produce polymer composite products, but the fibers used in the composites are short in the form of particles or powder (Figure 5).

• In a typical injection molding process, fiber composites in the form of pellets are fed through a hopper, and then they are conveyed by a screw with a heated barrel, as shown in Figure 5.
• Once the required amount of material is melted in a barrel, the screw injects the material through a nozzle into the mold where it is cooled and acquires the desired shape.
• Injection molding is found to be very effective for thermoplastic encapsulations of electronic products required in medical industries.
• Improvement in fiber-matrix compatibility and uniformity in the dispersion of fibers in the matrix material is achieved during the surface treatments of biocomposites [70,71].
Resin transfer molding: RTM is an established composite manufacturing process that is normally used to fabricate automotive and aircraft components. In this process, fiber reinforcement, either long or woven fibers is initially cut out using a template and a knife or scissors. These reinforcements called performs are bound employing a thermoplastic binder and then placed inside the mould cavity in the closed mould process. Resin is being transferred into the mould cavity by means of a variety of equipment such as by pressure or vacuum. Resins that are normally used in this process include polyester, epoxy, vinyl ester, and phenolic [69] (Figure 6).

Salim et al. used RTM to develop composite specimens for nonwoven kenaf fiber mat reinforced epoxy composites. Kenaf fiber mats were prepared after undergoing a series of processing such as opening, carding, cross lapping, and needle punching processes. Stitching density was optimized to study its effects on the mechanical properties of the composites. Besides, the effects of fiber loading on mechanical properties were also investigated. The mechanical properties improved when the kenaf mat in composites was stitched compared to unstitched kenaf mat composites.
Advance manufacturing process
The emerging nanotechnology has provoked researchers to find out new nano-scale fiber manufacturing techniques for composite materials. An electrostatic fiber manufacturing technique is called electrospinning uses electrical forces to generate continuous fibers of two nanometers to several micrometers. Polymer solution ejected through spinneret forms a continuous fiber, which is collected at the collector shown in Figure 7. It serves enhanced physical and mechanical properties, flexibility over process parameters, high surface area to volume ratio, and high porosity; therefore, it finds potential in diverse fields of biomedical applications such as wound healing, tissue engineering scaffolds, drug delivery, as a membrane in biosensors, immobilization of enzymes, cosmetics, etc. [72,73].

Automated manufacturing techniques
Filament winding: It is a continuous process that leads to selfautomation to reduce cost. Filament winding is useful to create axisymmetric, as well as some non-axisymmetric, composite parts, such as pipe bends. Driven by several pulleys, continuous prepreg sheets, rovings, and monofilament are made to pass through a resin bath and collected over a rotating mandrel, as displayed in Figure 8. Then, after applying sufficient layers, the mandrel, which has the desired shape of the product, is set for curing at room temperature. Recently developed robotic filament winding (RFW) technique is provided with an industrial robot equipped with a feed and deposition system. It yields advantages over process control, repeatability, and manufacturing time by replacing a human operator [74-77].

Biocomposites
Fibers provide strength and stiffness and act as reinforcement in fiber-reinforced composite materials; ultimately the properties of a composite are governed by the inherent properties of these fibers. Careful selection of the reinforcing fibers and matrix polymers, in light of the intended application, is the first step in obtaining a composite with the desired properties. Natural fibers are of basic interest since they can be functionalized and also have advantages from the point of view of weight and fibermatrix adhesion, specifically with polar matrix materials. They have good potential for use in waste management due to their biodegradability and their much lower production of ash during incineration. Nevertheless, the properties of a bio-composite may be controlled and indeed enhanced by altering those factors that control composite properties, namely fiber architecture and the fiber–matrix interface. The natural fiber reinforced polymer composite’s performance depends on several factors, including fibers chemical composition, cell dimensions, microfibrillar angle, defects, structure, physical properties, and mechanical properties, and also the interaction of a fiber with the polymer. To expand the use of natural fibers for composites and improved their performance, it is essential to know the fiber characteristics [23,78].
Physical characterizations
The physical properties of each natural fiber are critical and include the fiber dimensions, defects, strength, and structure. Several physical properties are important to know about for each natural fiber before that fiber can be used to reach its highest potential. Fiber dimensions, defects, strength, variability, crystallinity, and structure must be taken into consideration.
Knowledge about fiber length and width is important for comparing different kinds of natural fibers. A high aspect ratio (length/width) is very important in cellulose-based fiber composites as it indicates possible strength properties. Fiber strength can be an important factor in selecting a specific natural fiber for a specific application. Changes in the physical properties can be due to differences in fiber morphology. Major differences in the structure such as density, cell wall thickness, length, and diameter result in differences in physical properties. It is also interesting to note that the morphology of the land plant fibers is very from different that of water plant fibers. In between fiber qualities, the cellulose content and spiral angle is differed from fiber to fiber and also in a single fiber design.
Crystallinity values of natural fibers vary in different parts of the plant. The crystallinity tends to decrease as the plant matures, but the difference between bast and core fibers is inconclusive. The permeability of the kenaf core is highest and is closely followed by cotton [78] (Table 3).
Table 3: Physical characteristics of bio-fibers [78].

Mechanical properties
It is important to be knowledgeable of certain mechanical properties of each natural fiber to be able to exploit the highest potential it. Among these properties are the tensile, flexural, impacts, dynamic mechanical and creep properties.
Drying of the fiber before processing is necessary because moisture on the fiber surface acts as a debonding agent at the fiber-matrix interface. Additionally, because of water evaporation during the reaction, voids appear in the matrix (thermosets have a reaction temperature above 100°C). Both aspects lead to a decrease in the mechanical properties of biocomposites [79].
Tensile properties: The tensile properties are among the most widely tested properties of natural fiber-reinforced composites. The fiber strength can be an important factor regarding the selection of a specific natural fiber for a specific application. A tensile test reflects the average property through the thickness, whereas a flexural test is strongly influenced by the properties of the specimen closest to the top and bottom surfaces. The stresses in a tensile test are uniform throughout the specimen cross-section, whereas the stresses in flexure vary from zero in the middle to the maximum in the top and bottom surfaces.
Under tensile load, composites with efficient stress transfer show higher strength, higher stiffness, and lower strain at break than those with a weak interface. Rao et al. investigated the tensile properties of bamboo, date palm, abaca, oil palm, sisal, coir, and vakka fibers regarding their fiber cross-sections, atmospheric moisture content, and density. Khan, Ruhul A. et al. showed the value of TS is found to be 59.3MPa for 40% jute-containing composite which gained 77.17% increase in TS, respectively, then those of PVC matrix. TSs of 20% and 60% jute-containing composites are found to be 39.83 MPa and 51.13 MPa, respectively [79-81].
Flexural properties: Flexural stiffness is a criterion of measuring deformability. The flexural stiffness of a structure is a function based upon two essential properties: the first is the elastic modulus (stress per unit strain) of the material that composes it; and the second is the moment of inertia, a function of the crosssectional geometry.
Biocomposites were fabricated from chopped hemp fiber and cellulose ester biodegradable plastic via two different processes: powder impregnation by compression molding and an extrusion process followed by an injection molding process. Fabricated through process extrusion followed by injection molding process of hemp (30 wt%) fiber-reinforced cellulose acetate biocomposite exhibited flexural strength of 78 MPa and modulus of elasticity of 5.6 GPa as a contrast to 55 MPa and 3.7 GPa for the corresponding hemp fiber/PP based composite. The corresponding biocomposites showed superior flexural strength and modulus values during the extrusion and ensuing injection molding processes, as compared to biocomposites powder impregnated through the compression molding process [82].
Impact properties: Impact strength is the ability of a material to resist fracture under stress applied at high speed. Biofiber reinforced plastic composites have properties that can compete with the properties of glass fiber thermoplastic composites, especially concerning specific properties. However, one property, namely the impact strength is often listed among the major disadvantages of biofiber reinforced composites. In recent years, the development of new fiber manufacturing techniques and improved composite processing methods along with enhancement of fiber/matrix adhesion has improved the current situation somewhat. The impact strength of the bamboo fiber/PLA composites decreased after the addition of bamboo fiber. The impact strength of treated fiber-reinforced composites improved after the addition of treated fibers. Good fiber/matrix adhesion provides an effective resistance to crack propagation during impact tests. The impact strength increased significantly (33%) for silane treated bamboo fiber/PLA composites compared to untreated bamboo fiber/PLA composites with 30 wt% fiber content [83] (Table 4&5).
Table 4: Mechanical properties of different natural fibers and traditional fibers [3,4,84-89].

Table 5: Variation of mechanical properties of various natural fiber-reinforced polymer composites [90-92].
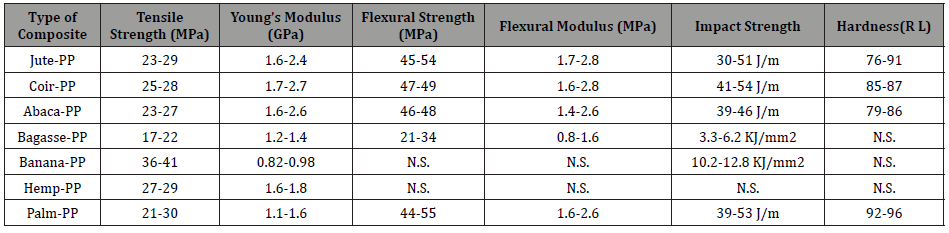
Tensile, flexural, and impact properties are the most commonly investigated mechanical properties of natural fiber-reinforced polymer composites. Impact strength is one of the undesirable weak points of these materials in terms of mechanical performance. Besides these tensile, flexural, and impact properties, the longterm performance (creep behavior), dynamic mechanical behavior, compressive properties are also investigated for natural fiber composites. To improve performance to the desired level, still much work is to be done considering fiber processing, non-linear behavior, fiber-matrix adhesion, fiber dispersion, composite manufacturing with optimized processing parameters [90-92].
Chemical properties
The chemical composition varies from plant to plant, and within different parts of the same plant. It also varies within plants from different geographical regions, ages, climate, and soil conditions. The chemical properties are influenced by the fiber growth time (days after planting), the botanical classification of the fiber, and the stalk height. The chemical composition can also vary within the same part of a plant. Both the root and stalk core have a higher lignin content than that of the fibers.
The chemical composition of the natural fibers, which varies depending on the plant from which they are derived, consists mainly of cellulose (50-70 wt%), hemicellulose (10-20 wt%), lignin (10-30 wt%), and pectin and waxes in smaller amounts [8].
Material Selection for Bio-Composites and Its Importance
Selecting materials is an important procedure of selecting specific material properties from a cluster of defined nominees after the physical design structures have been determined. Or identifying materials after appropriate manufacturing processes, which will hold the dimensions, shapes, and properties desired for the product to perform its required function at the lowest cost. Material selection for a specific purpose is an important task since materials play a significant role during the whole product design process. It requires interdisciplinary efforts with experts from diverse backgrounds, depending on the product field of application. The materials selection process is considered the main step of engineering design. Materials are responsible for function, shape, and interaction with other components of the product, also the effect on customer selection decision [93-101].
Future Developments
Developments and new trends for biocomposites have occurred as a result of the enhancement of fiber selection as well as polymer-fiber evaluation methodologies. This encourages all of the treatment and interfacial engineering in addition to composite processing. Hence, it is necessary to found practical motivations relative to environmental performance considering bio-materials. This in order would allow designers to make informed judgments without conducting exhaustive experimental work as well as waste time and effort. Such proposed methods may include elaborating more desired characteristics of bio-composites constituents that designers have to take into consideration for sustainable designs to enhance achieving better performance for the future [78].
Conclusive Remark
Researchers and investigators found many problems associated with the utilization of plastic-based products such as increasing costs and most importantly its negative impact on the environment. Therefore, researchers have recently discovered and focused their attention on biocomposites. Petroleum-based materials are replacing day by day by the development of biocomposites and also an alternative solution of synthetic fibers-based plastic composite products and generating more economical opportunities for the civil engineering sector. Further multidisciplinary research in the fields of engineering, agriculture, biology, and economy along with government support through education, multiple training programs, and giving tax reductions and breaks. Most importantly, eco-friendly or, environmentally friendly biocomposites from plant-derived fibers would be the blessing materials of this century as a solution to the increased environmental threats. Most research works have been carried out to study the potential uses of natural fibers for various technical applications. Among the most popular natural fibers; jute, hemp, flax, sisal, ramie, and kenaf fibers were extensively researched and employed in different applications. But in recent days, abaca, pineapple leaf, coir, oil palm, bagasse, and rice husk fibers are gaining interest and importance in both research and applications due to their specific properties and availability. Multidisciplinary steps for the manufacturing process are required to produce high-quality natural fibers that contribute to the cost of high-performance natural fibers as well as the improved mechanical properties of the composites. Natural fibers or, Biofibers or, lignocellulosic fibers surface modifications, development of bio-plastic as a suitable matrix for composite material processing and fabrication techniques play vital roles in designing and engineering biocomposites of commercial interest. Biocomposites reinforced with natural fibers and/or biopolymers are predestined to find more and more applications in the near future, especially in Europe, where pressure from both legislation and the public is rising. Biocomposites in building materials offer several significant advantages such as are cheap, lightweight, biodegradable, environmentally friendly, bio-renewable, and more durable. Several studies are examined, reviewed, and highlighted in this review paper regarding the importance of fiber-reinforced biocomposites as well as fabrication methods, physical and mechanical properties of biocomposites with future developments. Further research is required to overcome some obstacles such as inadequate toughness, moisture absorption, and reduced longterm stability for outdoor applications. Efforts to develop fiberreinforced biocomposite materials with improved performance for global applications are an ongoing process. To get a large market share and profits, need to manufacture fiber-reinforced biocomposites to the industries in a wide range.
To read more about this article...Open access Journal of Modern Concepts in Material Science
Please follow the URL to access more information about this article
To know more about our Journals...Iris Publishers
To know about Open Access Publishers
No comments:
Post a Comment