Authored by Jing Ye*,
Introduction
Cerebral venous thrombosis (CVT) is a relatively rare cerebrovascular disease which is accounting for 0.5-1% of all strokes and most commonly seen in young women [1,2]. After early anticoagulation treatment with heparin, parenchymal damage can be largely recovered in most of CVT patients. However, serious or life-threating consequence still happens in some CVT patients [3,4]. As such, it is important to identify the CVT patients who are at high risk of developing adverse clinical outcome. Few studies reported the potential risk factors for adverse clinical outcome of CVT. An animal experiment conducted by Nakase et al showed that regional cerebral blood flow (CBF) and oxygen saturation could predict clinical outcome of CVT [5]. It is well demonstrated that the extent of parenchymal damage can be evaluated by CBF measurement [6]. Gupta et al utilized Computed tomography perfusion (CTP) to measure the cerebral perfusion for 20 CVT patients and found that the relative CBF (rCBF) of the parenchymal abnormalities to contralateral regions in the contralateral hemisphere was significantly associated with adverse clinical outcome [7]. However, most of previous studies utilized CTP with administration of iodine-based contrast agent which is not applicable for patients with allergy to iodine or renal dysfunction. Arterial spin labeling (ASL) magnetic resonance perfusion imaging technique has become an alternative approach for assessing cerebral perfusion in clinical settings due to its advantages of no radiation and contrast agent free but similar performance with CTP [8]. Most recently, Furuya et al successfully evaluated the therapeutic course of 2 CVT patients by serial ASL [9].
Although routine MRI combined with magnetic resonance venography (MRV) has been considered as the gold standard for the diagnosis of CVT [10], the thrombosis in cortical veins and bridging veins could not be clearly displayed. In CVT patients, thrombosis can involve cortical and bridging veins and subsequently induce parenchymal damage and worsen the clinical condition due to the reduced perfusion, such as venous infarction and hemorrhage transformation [5,11,12]. The alternation of perfusion changes in CVT patients may reflect both venous congestion and the reduction of cerebral blood flow. Previous studies on CVT patients which involves cerebral perfusion information all showed perfusion abnormalities of parenchymal lesions [7,9,13,14]. Therefore, perfusion imaging may be helpful for the diagnosis and localization of CVT. The purpose of this study was to investigate the characteristics of cerebral perfusion by ASL and their predictive value for short-term clinical outcome in CVT patients.
Materials and Methods
Study population
Patients who were diagnosed with CVT by contrast-enhanced magnetic resonance venography (CE-MRV) were enrolled in this study. Routine brain MR imaging and ASL imaging for brain were performed for each subject after recruitment before treatment. The exclusion criteria are: 1) history of cerebral venous thrombosis in the past 6 months; 2) cerebral neoplasm; 3) conditions which can alter the perfusion parameters, such as intracranial stenosis and arteriovenous malformations. This study was approved by local institutional review board and written informed consent was obtained from each patient.
MR Imaging protocols
All MRI examinations were conducted on 3T MRI scanner (GE Medical Systems, Discovery 750 and 750W, Milwaukee, USA) with an 8-channel receiving head coil. All patients were well trained before MR examinations to keep their heads still during the scan. CE-MRV was performed using GRE sequence, parameters as follows: repeat time (TR)/echo time (TE) 3.5/1.4 ms, field of view (FOV) 240 × 240 mm2, flip angle 20°, slice thickness 2 mm. Routine brain imaging protocol was acquired with the following sequences: T1-weighted (T1W) (TR/TE 2534/19 ms, FOV 240 × 240 mm2, slice thickness 5 mm, and number of slices 22); T2-weighted (T2W) (TR/TE 5325/101 ms, FOV 240 × 240 mm2, slice thickness 5 mm, and number of slices 22); and Diffusion weighted imaging (DWI) (TR/TE 4488/79.5 ms, FOV 240 × 240 mm2, slice thickness 5 mm, and number of slices 22). Perfusion-weighted images were obtained by acquiring 3D pCASL sequence. In addition to the background suppression pulses within the slab, inferior saturation pulses were applied to suppress the inflowing arterial blood spins. The imaging parameters for 3D pCASL were as follows: 3D spiral fast spin echo (FSE) sequence, TR 4844 ms, TE 10.5 ms, FOV 240 × 240 mm2, slice thickness 4 mm, slice gap 0 mm, number of slices 36, 512 sampling points on eight spiral arms, spatial resolution 3.64 mm, bandwidth 62.5 kHz, NEX 2, post labeling delay (PLD) 2025 ms, and labeling duration 2000 ms. No motion correction and slice timing correction were performed.
Clinical follow-up
The follow-up MRI was conducted for brain within 3 weeks after anticoagulation treatment. The routine brain imaging protocol was performed for the follow-up examination. The adverse clinical outcome in this study was recorded which is defined as: 1) enlargement of parenchymal lesions; or 2) cerebral hemorrhagic transformation.
MR image analysis
The ASL perfusion images were transferred to Advantage Workstation 4.6 (GE medical systems) with dedicated software of 3D-ASL with single compartment model assumed. Subsequent quantifications were performed according to the following equation:
where T1b was defined as for the T1 of the blood (1600 ms), T1g as the T1 of the grey matter (1200 ms), α as the labeling efficiency (0.8), λ as the cortex–blood partition coefficient (0.9), tsat as the time of saturation performed before imaging (2000 ms), τ as the labeling duration (2025 ms), and PLD as the postlabeling delay time [15].15 In addition, proton density weighted images, denoted as PD here, were directly acquired during ASL image acquisition. A moderate image smoothing was applied by using 3 × 3 convolutional kernel.
Cerebral blood flow (CBF) was measured by 2 experienced radiologists (>3 years’ experience in neuroimaging). The regions of interest (ROIs) were placed at the areas of parenchymal abnormalities characterized by hyperintense on T2W and DWI images and the contralateral regions (normal reference) in contralateral hemisphere. The CBF of ROIs was measured for 2 times by one radiologist. The mean values of CBF and the ratio of CBF (rCBF = CBF of parenchymal abnormalities / CBF of contralateral regions) between the measurements of 2 times were calculated for statistical analysis.
Reproducibility of ASL measurements
All patients were selected for testing the inter-observer and intra-observer reproducibility in measuring CBF. Two radiologists independently measured the CBF values of the whole ROIs of parenchymal abnormalities and contralateral normal hemisphere on ASL images for all patients. One radiologist performed a repeat measurement of the CBF values of the whole ROIs in above cerebral regions on ASL images with a time interval of 3 months to minimize the memory bias.
Statistical analysis
All statistical analyses were performed by SPSS (V.19.0; SPSS Inc, Chicago, Illinois, USA). Kolmogorov–Smirnov test was applied to estimate the normality of all the measurements. The continuous variables were presented as mean ± standard deviation (SD) and the categorical variables were presented as frequency. The absolute CBF values of grey matter, white matter, and whole ROIs were compared between parenchymal abnormalities and the contralateral regions with paired t test. Baseline clinical characteristics, cerebral absolute CBF values and rCBF were compared between patients with and without adverse clinical outcome using independent t test, Mann-Whitney U test, or Chi-square when appropriate. Logistic regression was used to calculate the odds ratio (OR) and 95% confidence interval (CI) of rCBF in predicting adverse clinical outcome before and after adjusting for confounding factors. Grubbs’ outlier test was performed before logistic regression. The receiver-operating-characteristic analysis was utilized to determine the area-under-the-curve (AUC) of rCBF in discriminating adverse clinical outcome. The intraclass correlation coefficient (ICC) and corresponding 95%CI of rCBF were calculated to determine the inter-observer and intra-observer agreement. A P value < 0.05 was considered as statistically significant.
Results
In total, 23 patients (mean age 42.09 ± 14.71 years; 14 females) with CVT were included in this study. Of the 23 patients, 17 (73.91%) presented with headache and 1 (4.35%) was postpartum at baseline. The potential causes of CVT of this study population included oral contraceptives for a long time (n=6), infections (n= 5), puerperium (n=1), nephrotic syndrome (n=1), and sicca syndrome (n=1). The potential cause for the rest 9 patients is unknown. During 3 weeks’ follow-up, 7 (30.43%) patients developed adverse clinical outcomes, including 4 (17.39%) patients with enlargement of parenchymal lesions and 3 (13.04%) patients with both hemorrhagic transformation and enlargement of parenchymal lesions.
Imaging characteristics on MRV and ASL images
On MRV images, CVT can be found in 15 (65.22%), 17 (73.91%), 5 (21.74%), and 3 (13.04%) patients in sagittal superior sinus, transverse and sigmoid sinus, straight sinus, and cortical veins, respectively. Of the 23 patients, 9 (39.13%), 12 (52.17%) and 2 (8.70%) were found to have CVT in 1, 2, and >2 regions, respectively. In this study population, all ASL perfusion abnormalities were localized adjacent to the occluded sinus. Of all 23 patients, 20 (86.96%) showed hypoperfusion and 3 (13.04%) showed hyperperfusion in parenchymal abnormalities compared to contralateral regions. Paired t test showed that the absolute CBF of the parenchymal abnormalities was significantly lower than that of contralateral regions (33.98 ± 13.52 mL/100g/min vs. 46.69 ± 14.10 mL/100g/ min, P = 0.003). There were no significant differences in the absolute CBF values of parenchymal abnormalities (37.21 ± 20.19 mL/100g/min vs. 26.79 ± 6.15 mL/100g/min, P = 0.200) between patients with good and adverse clinical outcome. Patients with adverse clinical outcome showed significantly lower rCBF (0.54 ± 0.17 vs. 0.83 ± 0.18, P = 0.002) compared to those with good clinical outcome (Figure 1&2).
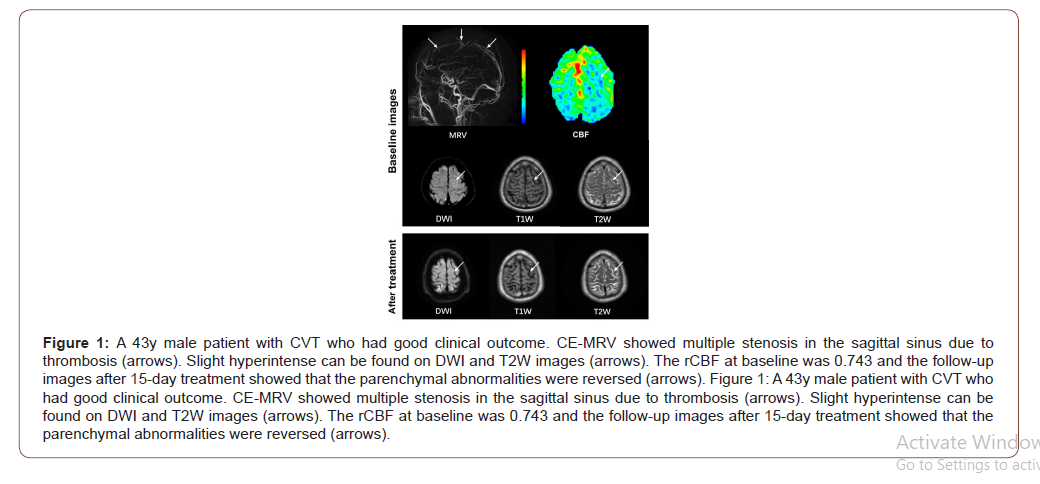
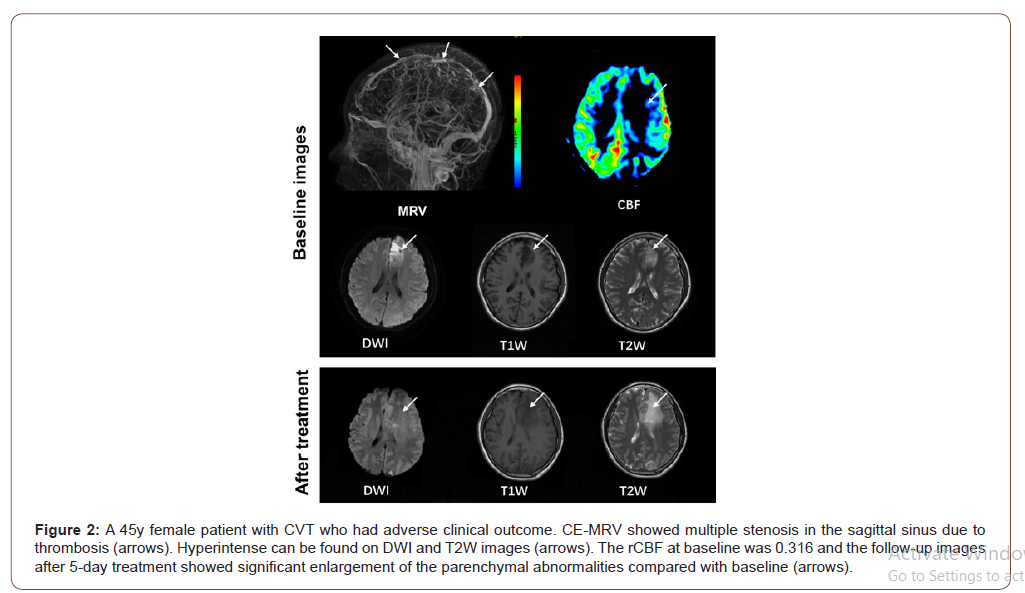
Association between rCBF and adverse clinical outcome
No outlier was found by Grubbs’ outlier test. After that, logistic regression analysis demonstrated that rCBF of the whole ROIs was significantly associated with adverse clinical outcome before (OR = 0.207, 95% CI 0.049-0.879, P = 0.033) and after (OR = 0.167, 95% CI 0.030-0.914, P = 0.039) adjusting for age and gender with increment of 10% of rCBF. ROC curve analysis indicated that patients with rCBF < 65.2% (optimal cut-off value) were more likely to have adverse clinical outcome (AUC = 0.893, sensitivity 87.5%, specificity 85.7%, Figure 3).
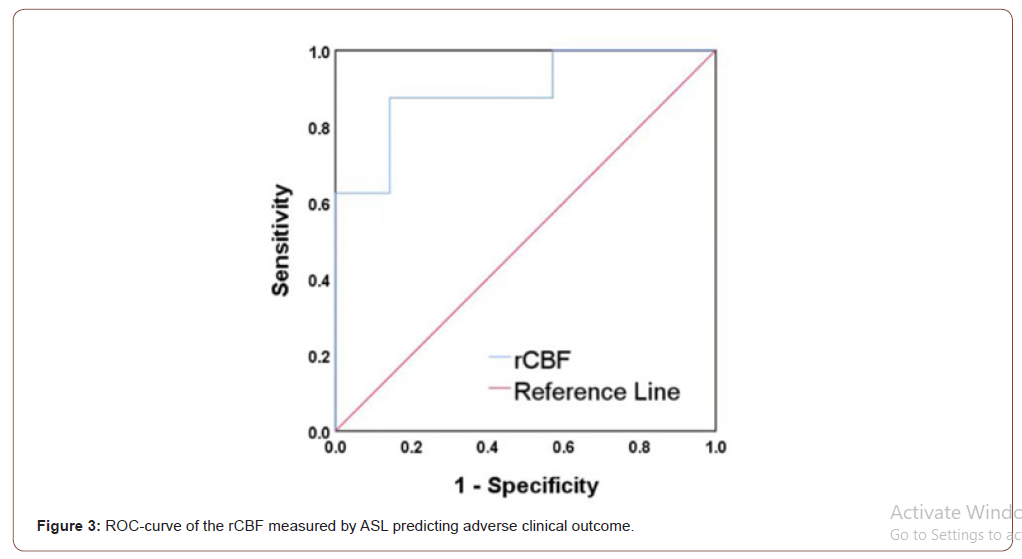
Reproducibility of ASL measurements
Excellent intra-observer (ICC, 0.964; 95%CI, 0.917-0.985) and inter-observer (ICC, 0.936; 95%CI, 0.857-0.972) agreements were found in measuring rCBF using ASL.
Discussion
This study investigated the characteristics of cerebral perfusion in cerebral parenchymal abnormalities of CVT patients before anticoagulation treatment using ASL and their predictive value for short-term adverse clinical outcome. We found that patients with adverse clinical outcome had significantly lower rCBF compared with those without. Logistic regression analysis revealed that rCBF was independently associated with adverse clinical outcome. Our findings suggested that the perfusion determined by ASL before anticoagulation treatment might be an effective predictor for shortterm adverse clinical outcome in patients with CVT. In this study, most of the CVT patients (20/23) had lower perfusion in parenchymal abnormalities compared to contralateral regions. Similar results were reported in a study by Kang et al (10/13) [13]. Even though the cerebral collateral pathway is sufficient, cerebral venous thrombosis will lead to intracerebral venous congestion and the increase of venous pressure, which may also lower the perfusion pressure [16,17]. In our study population, 3 patients showed relative hyperperfusion in the parenchymal lesions which may be due to the accumulation of acidic metabolites that result in vasodilation in the abnormal area or the physiological compensation of reserved capillaries in the early period of CVT.
In this study, we found that the baseline rCBF in patients with adverse clinical outcome was significantly lower than that in those patients without and lower rCBF could effectively predict adverse clinical outcome in CVT patients. Nakase et al reported that the extent of CBF reduction reflected the subsequent parenchymal damage in CVT patients [6]. The causes of arterial stroke and cerebral venous infarction are different. In arterial stroke, the occlusion of feeding artery directly leads to the reduction of cerebral perfusion. In contrast, cerebral venous infarction was commonly caused by cerebral venous thrombosis [18]. The reduced CBF may lead to functional disturbance of brain tissue in both cerebral events [19]. Pathophysiologically, in arterial stroke, decrease in CBF can induce the lack of glucose and oxygen, in turn, the energy failure leads to a cascade of the depletion of adenosine triphosphate (ATP) and a decrease in sodium-potassium adenosine triphosphatase (Na+-K+ ATPase) activity [20]. In contrast, the fact of pathophysiology of CVT is a multistep evolution and the time course of parenchymal damage is prolonged, which can persist for a long time but still may be reversible [12,19]. Whether the damage of cerebral parenchymal is reversible depends on the extent of reduction in cerebral perfusion. It might be plausible that the anticoagulation drug does not get to be delivered to the thrombus effectively when perfusion is lowered to a certain degree. A previous study on arterial stroke conducted by Shimosegawa et al showed that completed infarction induced by 40% reduction of CBF, but no such threshold has been reported in CVT patients [21]. Therefore, we assumed that this threshold may be also suitable for patients with CVT. Gupta et al determined the pre-treatment measurements in the lesions and contralateral regions by CTP in CVT patients and found that rCBF was the best parameter to evaluate the clinical outcome with the optimal rCBF cut-off value of 60.5% (AUC=0.755, sensitivity 68.2%, specificity 66.7%) [7]. This optimal cut-off value is consistent with the results in arterial stroke to some extent. The optimal cut-off value of rCBF in the present study is slightly higher than the literature report which might be due to the limited sample size.
A single PLD of 2000 ms was recommended as a standard clinical protocol for adult population, which is a robust and straightford means of obtaining reliable CBF images [22]. However, the difference in individual PLD and inappropriate PLD will attenuate the accuracy of CBF measurement. Both the arterial transit time (ATT) and CBF can be accurately estimated when serial ASL images were acquired with multiple PLDs [22,23]. Few previous studies utilized ASL to assess cerebral perfusion in CVT patients. There might be different in the mechanism of cerebral ischemia between arterial occlusion and venous congestion. Furuya et al conducted ASL imaging with both PLDs of 1000 ms and 2200 ms, despite the scan parameters were different from the present study , they found that shorter PLD of 1000 ms couldn’t reflect the different perfusion between parenchymal abnormalities and contralateral regions in CVT patients [9]. Accordingly, a moderate PLD of 2025 ms was used in the present study. This may be a limitation of this study. It might be ideal to use multiple PLD times in ASL imaging for accurate quantification of CBF and transit time in future studies. Beyond single PLD, other limitations should be noted in this study. First, we measured rCBF by drawing the ROIs according to the hyperintense on DWI which may represent the core area of infarction. Although a study by Gupta et al demonstrated that there was no significant difference in cerebral perfusion between core and periphery regions [7] which may represent penumbra in parenchymal abnormalities, it might be interesting to evaluate the characteristics of CBF in periphery regions of parenchymal abnormalities. Second, in the present study, only the most severe slice with maximum area of ischemia was selected for measuring rCBF in each patient. The selected slice may not represent the overall lesion. Finally, the sample size of the present study is limited. Larger patient population is suggested to be included in future studies.
Conclusion
In conclusion, the cerebral perfusion determined by ASL in CVT patients is independently associated with short-term adverse clinical outcome, suggesting that baseline cerebral perfusion might be an effective predictor for prognosis in CVT patients.
To read more about this article....Open access Journal of Neurology & Neuroscience
Please follow the URL to access more information about this article
To know more about our Journals...Iris Publishers
To know about Open Access Publishers
No comments:
Post a Comment