Authored by Antonio Rueda-Domínguez*,
Abstract
Dendritic cells, via their antigen-presenting function, play a fundamental role in the antitumour immune response. In patients with lymphoma, dendritic cells activity has been associated with tumour progression and prognosis, and hence cause-specific survival. Moreover, some gene expression signatures with prognostic value for follicular lymphoma patients are dendritic cell specific. Vaccines based on the specific stimulation of dendritic cells have recently been developed, with very promising results in animal models and in early clinical trials, with an objective response rate of over 30%. Therefore, immunotherapy based on dendritic cells may become an integral part of advanced treatment for patients with lymphoproliferative syndrome.
Keywords:Tumour microenvironment; Dendritic cells; Lymphoma; Dendritic cell vaccines
Introduction
The immune system plays a key role in the control and survival of tumour cells, and the investigation of various immunotherapy strategies is currently revolutionizing cancer treatment. In this respect, one of the most promising strategies is cellular immunotherapy to generate specific antitumour T cells and dendritic cell-based vaccines [1-4]. Although dendritic cells (DCs) are the main antigen-presenting cells, their function and biology are still largely unknown. These cells are activated after phagocytosis and antigen processing, and are capable of stimulating naive T cells, thus playing a crucial role in promoting the host’s immune response by means of appropriate tumour antigens [5-9]. The clinicalbiological and prognostic relevance of several non-neoplastic cell types within the tumour microenvironment has been extensively studied in lymphomas. However, much less is known about the role of DCs as possible mediators of immune escape and/or tumour progression [10,11].
DCs and natural killer (NK) cells are the main effector cells of the innate immune system. Dendritic cells stimulate the activation of NK cells, improving interferon-γ (IFN-γ) secretion and increasing NK cell proliferation and antitumour activity. This interaction fosters the interconnection of innate and adaptive immunity, thus enhancing the quality of the immune response, enabling the recognition and elimination of microbial pathogens and transformed cells and inducing the initiation and regulation of the adaptive immune response [12,13]. This phenomenon has been observed, among other cases, in patients with localised (stage II/IIIA) breast cancer, for whom treatment with vaccines based on dendritic cells has facilitated an increase in the number of NK cells in peripheral blood, an expansion of tumour-specific CD8+ T cells and their differentiation into IFN-γ-producing effector cells [14-18].
The high toxicity of standard cancer treatment has driven the search for increasingly specific targeted antitumour therapies. DCs that are genetically modified to express specific proteins offer a promising line of treatment against infections and neoplastic processes [19], heightening the capacity to stimulate the production of CD4+ and CD8+ T cells against specific antigens [12, 20-24]. This outcome is corroborated by the correlation between the immune response induced by vaccines based on dendritic cells and increased patient survival. In recent years, dendritic cell-based immunotherapy has been widely investigated in patients with different types of haematological malignancies [25] and has obtained very promising results with respect to follicular lymphoma [26,27]. Furthermore, high expectations have been raised by the combination of immunomodulatory drugs and vaccines against tumour antigens [28,29]. In the following sections, we review the role of DCs in the progression of lymphoproliferative syndromes and consider their potential use in the treatment of these neoplasms.
Dendritic Cells: Characteristics, Function and Biology
Dendritic cells, derived from CD34+ pluripotent stem cells, are large, irregularly shaped cells with numerous membrane protrusions, prominent mitochondria, endosomes and lysosomes, all of which are involved in the identification, uptake and processing of antigens [9]. These cells present a number of surface antigens that distinguish them from macrophages and monocytes, such as CMRF-44, CD83, adhesion molecules (CD11a, CD11c), co-stimulatory antigens (CD80, CD40 and CD86) and molecules of the major histocompatibility complexes (MHC) I and II [1]. Following antigen absorption and processing, DCs mature and migrate through the tissues to the secondary lymphoid organs. This maturation occurs with the recognition of pathogen associated molecular patterns (PAMPS), binding to Toll-like receptors on the cell surface. Recognition of these receptors triggers a cascade of intracellular signals that activates nuclear factor jD, resulting in dendritic cell maturation with an increased expression of costimulatory molecules, adhesion molecules and cytokines. In the secondary lymphoid organs, these mature DCs with the processed antigen activate the T cells in association with the molecules of the MHC. This activation also requires a series of co-stimulatory molecules, consisting of the CD8 (B7.1) and CD86 (B7.2) expressed by the antigen-presenting cell, which bind to the CD28 and CD40 ligands present on the surface of the T cells [9]. These costimulatory molecules determine whether the T cells induce a type 1 (inflammatory) or type 2 (humoural) immune response, or one derived from the Th17 cells producing Granulocyte Colony- Stimulating Factor (G-CSF) and Granulocyte Macrophage Colony- Stimulating Factor (GM-CSF) [1,2,30,31].
DCs overexpress these co-stimulatory molecules only if they recognise signals that indicate that the cell is under stress, such as the presence of inflammatory cytokines, high levels of pathogens, and prostaglandins. The inflammatory cytokines that play a critical role in antitumour activity are tumor necrosis factor α (TNF-α) and IFN-γ [32]. In contrast, TGF-β favours tumour progression and the development of metastases [32,33]. IL-15 is the most important inflammatory cytokine in cell activation, facilitating the survival of memory CD8-α T cells. IL-15 activated dendritic cells present antitumour potential, which has been observed in various breast cancer cell lines in vitro [34,35]. If the antigen is recognised without binding to a Toll-like receptor, the DCs will remain immature. In this case, the T cells will not be activated, and there may be a proliferation of regulatory T cells (Tregs) [9]. DCs, thus, may induce a lack of response (or anergy), through the expansion of Tregs, negatively regulating the immune response [31,36]. Figure 1 reflects the mechanisms by which dendritic cells participate in antitumor activity (Figure 1).
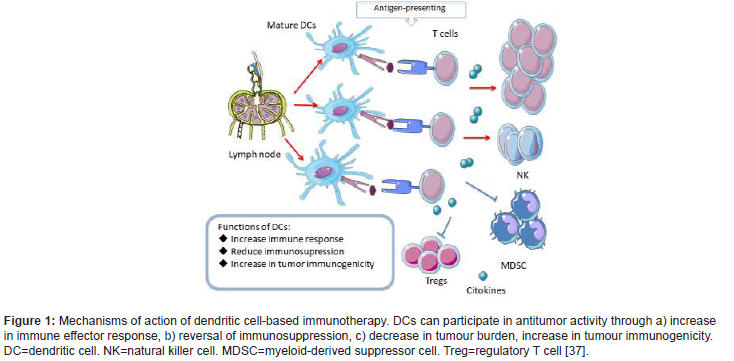
Various subtypes of DCs can be found in peripheral blood, including conventional dendritic cells (cDC) CD11C+, also termed myeloid dendritic cells (mDC), consisting of CD1C+ (mDC1) or CD141+ (mDC2) cells, and plasmacytoid dendritic cells (pDC) CD11C-, composed of CD123+ or CD303+ cells [38-41]. Table 1 shows the different subtypes of dendritic cells. mDC play a fundamental role in pathogen detection, in the presentation of antigens to T lymphocytes and in the specific stimulation of CD4 + and CD8+ T cells. pDC, on the other hand, exert strong antiviral activity through the production of IFN-1 and contribute to the antitumour response through a synergistic interaction with the mDC [42]. One type of partially mature dendritic cells is formed from human CD14+ monocytes in the presence of GM-CSF and IFN-α (IFN-DC). These cells favour the Th1-type cellular response and stimulate the immune response of CD8+ T cells [43-46] (Table 1).
Table 1: Dendritic cell subsets [47].
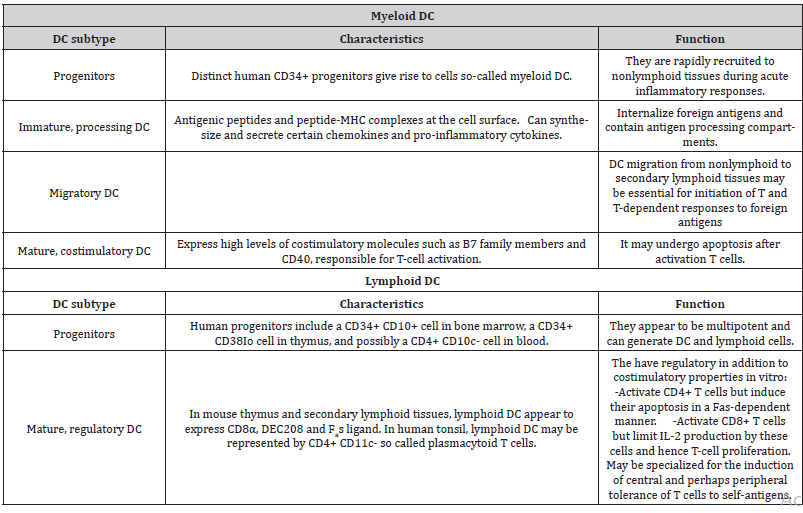
Role of Dendritic Cells in Lymphomas
Many studies have corroborated the potential of DCs for use as prognostic biomarkers in lymphoproliferative syndromes, or in some cases even for supporting the diagnosis. For example, Fiore et al. (2006) analysed DCs in 55 patients with non-Hodgkin lymphoma (NHL) and in 33 reactive lymph nodes, observing that the presence of these cells in the reactive lymph nodes was greater than in the cases of NHL, while the pDC/mDC ratio was similar. The conclusion drawn was that the presence of DCs crucially induces a specific antitumour immune response. The lower presence of DCs in patients with NHL explains the decreased expression of CD62L and CCR7 (dendritic cell receptors). With NHL, phenotypic alteration and a decreased presence of DCs in the tumour infiltrate may contribute to the loss of antitumour immune control in these patients [48]; this outcome has been documented in a variety of haematologic malignancies, including chronic lymphocytic leukaemia, hairy cell leukaemia [49], acute lymphoblastic leukaemia [50], multiple myeloma [51,52], chronic myeloid leukaemia [53-56], classical Hodgkin lymphoma (cHL) [56] and other cancers [57], as well as inflammatory disorders such as systemic lupus erythematosus [58] and uveitis [59]. This immunosuppressive state of the tumour microenvironment could be secondary to the metabolic stress, hipoxia and cytokine secretion that can negatively affect DCs [57]. cHL is a monoclonal lymphoid neoplasm composed of mononuclear Hodgkin cells and multinucleated Reed-Sternberg cells (HRS) embedded in an overwhelming mix of inflammatory and immune cells that contribute to tumour expansion by providing survival signals and promoting immune escape [60,61]. The significant impact of the inflammatory microenvironment in cHL motivated a study of the role of DCs in 52 patients diagnosed with de novo cHL, of whom 50 were treated with the ABVD polychemotherapy regimen (doxorubicin, bleomycin, vinblastine, dacarbazine). The count of all DCs subtypes was lower in patients with cHL compared to healthy controls. Moreover, the mDC count was lower in patients with advanced stage disease compared to those with an earlier stage, both for mDC1 and for mDC2. In addition, the mDC2 count decreased in cases with bulky disease and extranodal disease. These data suggest that a lower presence of mDC2 in the tumor microenvironment is related to a more advanced stage. The preeminent role of mDC2 in antigen presentation and in the induction of antitumour immunity, with respect to other DCs subtypes [62–64] could explain these facts [62–64]. Low levels of mDC1, mDC2 and pDC have also been reported in patients with B symptoms. It is not clear why cHL is characterized by a lower presence of DCs in the tumor microenvironment and why an increase is observed during chemotherapy treatment [56].
In the case of follicular lymphoma (FL), a better understanding of the molecular events responsible for differences in patients’ survival could facilitate a more accurate method for stratifying risk, guiding treatment and suggesting new therapeutic approaches. This question is particularly important because prognostic models based on the clinical variables of routine clinical practice are not useful in determining the best initial treatment. From a cohort of 191 biopsies of treatment-naive FL patients, gene expression profiles were studied in a training cohort of 95 samples and validated in an independent cohort of 96 samples [65]. Two gene expression signatures were obtained (immune-response 1 and 2) capable of predicting survival at the start of treatment. These signatures were composed of genes related to the expression of non-malignant immune cells present in the tumour. The binary model obtained from the joint consideration of these two signatures was a better predictor of survival than one obtained from either signature alone. The survival results were grouped into four quartiles, with the following median periods of survival: quartile 1, 13.6 years; quartile 2, 11.1 years; quartile 3, 10.8 years; quartile 4, 3.9 years. The “immune response 1” signature included genes encoding T cell markers (CD7, CD8B1, ITK, LEF1 and STAT4) and also genes that are highly expressed in macrophages (ACTN1 and TNFSF13B). The “immune-response 2” signature, on the other hand, included genes expressed mainly in macrophages, DCs or both (TLR5, FCGR1A, SEPT10, LGMN and C3AR1) [65-71].
These findings suggest that the survival of patients with FL is correlated with the molecular profile of non-malignant immune cells present in the tumour at diagnosis (including DCs). This understanding provides us with a tool to investigate different aspects of the immune response in FL that could positively or negatively influence the evolution of the disease. The identification of genetic signatures related to the immune response might constitute a valuable basis for studying biomarkers related to different subpopulations of immune cells that could promote or hinder the survival of malignant lymphoid cell clones [65]. Primary cutaneous marginal zone B-cell lymphoma (PCMZL) is an indolent lymphoma with a five-year survival of 95-98% [72,73]. Histologically, it is composed of a proliferation of small, centrocyte-like lymphocytes, monoclonal plasma cells, reactive germ centres and small T cells [74]. It presents multiple clinical pathological characteristics that are common to cutaneous Bcell pseudolymphomas (CBCL). However, PCMZL can present high levels of CD123+ pDC in the tumour (100% of cases), unlike CBCL, where these infiltrates are much less extensive (64%). They are even less frequent in cutaneous centre follicular lymphomas (13%) and are totally absent from primary cutaneous diffuse large B-cell lymphoma, leg type. The presence of high intratumoural levels of pDC supports the diagnosis of PCMZL. Furthermore, the pDC in PCMZL lack the expression of CD68 whereas in the absence of cutaneous lymphomas the pDC express both CD123 and CD68 [75]. The presence of high levels of pDC in this lymphoma may explain the large numbers of T cells generally found there, which can account for up to 60% of the tumour cell component [76]. These data support the hypothesis that pDC and T and B cells are necessary for the development of PCMZL, especially pDC (which is present from very early lesions). Therefore, the use of treatment strategies targeting antigen-driven T-cells or even the pDC responsible for the stimulation of B cells could be very effective in the treatment of PCMZL [77].
The Role of Dendritic Cells in Treating Lymphoproliferative Syndromes
Recent studies have highlighted the potential role of DCs in the treatment of patients with malignant tumours. These cells can be loaded with specific tumour antigens that trigger an antitumour response by specific T cells (Figure 2). To achieve this, the dendritic cells are cultured with tumour peptides or proteins, or the tumour antigen is expressed through the inoculation of adenoviruses that transfer genetic material to encode these specific tumour antigens [9]. Dendritics cells are obtained from peripheral blood. To mobilize dendritic cells to the peripheral blood it is recommended to use GM-CSF. When the regimen includes GM-CSF, mobilization of DCs to peripheral blood is 3-5 times more effective [78]; this contrasts with NK cell mobilisation, which is just as efficient regardless of whether G-CSF, GM-CSF or GM-CSF followed by G-CSF is used [79]. In this respect, Avigan et al. (1999) [78] obtained a greater quantity of DCs when the peripheral blood DCs precursors in patients were mobilised with GM-CSF + G-CSF, compared with the use of G-CSF alone (Figure 2).
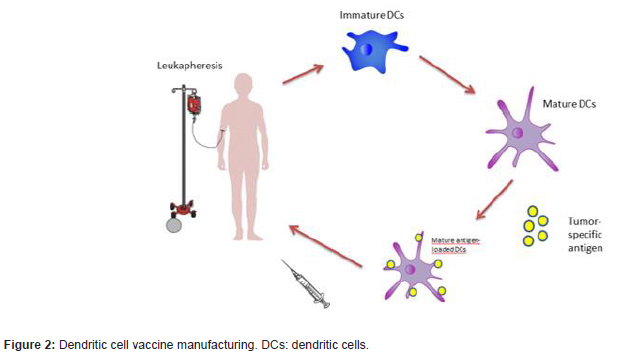
However, there are some limitations to the use of DCs as therapeutics agents, especially due to the scarcity of certain subgroups of DCs in peripheral blood. In consequence, most dendritic cell-based vaccines employ mDC differentiated from circulating monocytes in peripheral blood, due to the relative ease with which these monocytes can be obtained [80]. DCs differentiation can be induced from monocytes cultured with IL4 and GM-CSF [81] or from CD34 cells [82] cultured with a wide variety of growth factors such as GM-CSF + TNF-α, GM-CSF + SCF and IL4, TNF-α and ligand flt3 with TGF-β [51,83,84].
Most studies of dendritic cell-based vaccines in lymphoproliferative syndromes have been performed in patients with FL. This is the most common form of indolent lymphoma (accounting for 70% of the total) and is usually diagnosed at advanced stages of the disease [85]. The standard treatment is a combination of rituximab with chemotherapy followed by rituximab maintenance. Although most patients will relapse, over 80% of patients obtain long-lasting remission, this approach is complex, expensive and heightens both acute and long-term toxicity [86,87]. n general, subsequent lines of treatment are progressively less effective; thus, toxicity increases, and the patient’s quality of life deteriorates [88,89]. When relapses occur, most patients experience a greater risk of transformation and treatment-induced toxicity [85,88,90]. Therefore, effective long-term management is necessary. Recent studies have shown that dendritic cell-based treatment enables a specific immune response associated with sustained tumour regression in some patients with low tumour burden FL. The objective response rate with sustained remission (33-50%), as shown in Table 2, is clearly greater than that obtained in studies of solid tumours, which corroborates the sensitivity of FL to this treatment strategy [80] (Table 2).
Table 2:Main clinical trials with dendritic cell-based vaccines in follicular lymphoma.
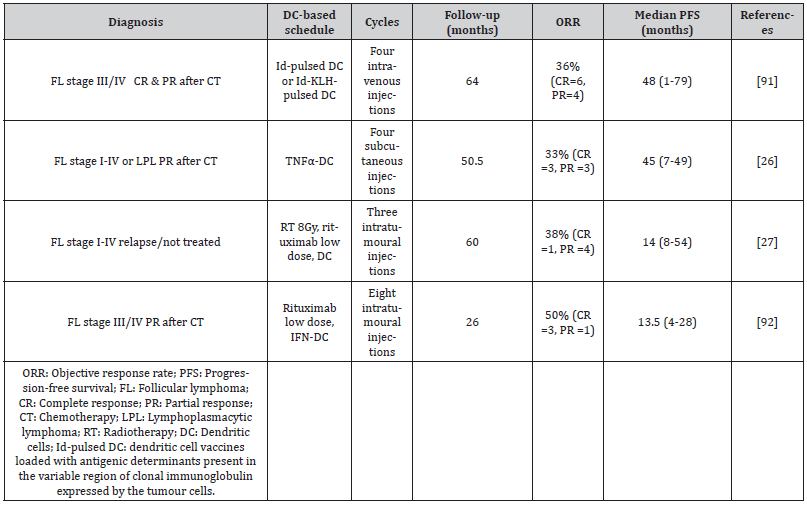
Timmerman et al. (2002) [91] studied 35 patients with relapsed or refractory FL, treated after chemotherapy with dendritic cell vaccines loaded with antigenic determinants present in the variable region of clonal immunoglobulin expressed by the tumour cells (Id-pulsed DC vaccination). Of the 18 patients with residual disease at the time of the vaccine, four had tumour regression and 16 remained progression-free at a median 43 months after chemotherapy. Six patients with progression after this vaccine received another with the Id- KLH protein (idiotype protein bound to the highly immunogenic transporter protein keyhole limpet hemocyanin). Tumour regression took place in three patients (two with complete response and one with partial response). These data suggest that Id-pulsed DC vaccination can induce a humoral and cellular immune response with lasting tumour regression, and that subsequent vaccination with ID-KLH can induce tumour regression despite apparent resistance to the initial Id-pulsed DC vaccination [91]. In another study, 25 patients in initial remission after cytoreductive chemotherapy received three monthly infusions of Idpulsed DC followed by a fourth, 2-6 months later. After vaccination, 23 of the 25 patients developed a T cell response and 36% of those with an evaluable tumour had an objective response to the vaccine [91]. Bendandi et al. (1999) [93], too, obtained excellent diseasefree survival, in 18 of 20 patients with FL treated with Id-KLH DC + GM-CSF, although this study was limited to patients who achieved a complete response after chemotherapy. These findings suggest that the response to Id-pulsed DC is more frequently obtained in patients presenting a complete response when the vaccine is received.
A second generation of vaccines combines Id-pulsed DC with chemokines, enabling the DCs to capture the antigen. Sakamaki et al. (2014) associated this vaccination strategy with lenalidomide, an immunomodulatory drug that reduces the number of systemic myeloid derived suppressor cells (MDSCs), and Tregs, thus improving tumour immunosuppression [94]. This approach is consistent with previous studies showing that lenalidomide inhibits the proliferation and function of Tregs [95,96] and increases the quantity of NK cells [97]. In a study by Di Nicola et al. (2020), 33% (6/18) of heavily pretreated and/or refractory patients with follicular and lymphoplasmacytic lymphoma obtained lasting clinical remission after repeated four-dose dendritic cell vaccination [26]. Another 33% remained stable, during a median follow-up of 50 months. Among the responders, NK cells were activated, which correlated with a reduction in the frequency of Tregs. Combining anti-tumour vaccines with immunomodulatory drugs is considered a very promising approach to boost the immunity of tumour-specific T cells and eradicate residual malignant cells. Dendritic cell-based strategies can safely be combined with radiation therapy, immune checkpoint inhibitors, immunomodulators and metronomic chemotherapy. However, clinical trials are still at a very early stage.
The optimal conditions for the culture of effective mDC is unknown, but a quick and easy method is to perform the culture in the presence of IFN-α and GM-CSF, which obtains partially mature and highly active mDC from peripheral blood monocytes (IFNDC) [46,98]. IFN, which presents as a signal of damage in cases of infection/inflammation, can initiate a cascade of differentiation of monocytes into dendritic cells, resulting in the activation of NK cells, the generation of a Th1 helper T lymphocyte immune response and the induction of a CD8+ T cell response towards both pathogens and neoplastic cells. The use of IFN-DC enables antitumour vaccines to be created endogenously [27,99,100]. In the phase I clinical trial of Cox et al. (2019), eight patients with relapsed or refractory FL were sequentially treated with the intranodal administration of low-dose rituximab followed by IFN-DC not loaded with tumour antigens. In this process, the first four cycles were administered every two weeks and the remaining four cycles, at four-week intervals. GMCSF and IFN-α facilitated the differentiation of blood monocytes to DCs, thus incorporating the tumour antigen and activating the CD8+ T cells faster than with conventional DCs and enhancing the antitumour immune response [45,101-103]. Among the eight patients, the overall response rate was 50% (4/8). Three patients obtained a complete metabolic response, and two maintained a remission (median follow-up 26 months, range 11-47). These results demonstrate the induction of a systemic response based on an abscopal effect and highlight the effectiveness of IFN-DC treatment in inducing a long-lasting clinical response, together with the ability to promote the stimulation of peripheral tumourspecific T cells, through the endogenous formation of an effective antitumour vaccine [92].
As concerns the association of radiotherapy and dendritic cell-based vaccination, radiotherapy promotes the cellular and molecular reprogramming of the tumour microenvironment, which in turn favours the maturation of DCs into effective antigenpresenting cells [104]. Kolstad et al. (2015) [27] studied fourteen patients with stage III/IV FL, in first-line treatment or in relapse, who were treated with 8 Gy radiotherapy in a specific lymph node región followed by the intranodal injection of rituximab at low doses (5mg) and cDC + GM-CSF. The treatment was repeated three times (weeks 1, 3 and 5), in a different node each time. A strong correlation was observed between the reduction in tumour area and the magnitude of the antitumour response of the CD8+ T cells generated. Five of the 14 patients (36%) obtained a clinical response, regardless of the tumour load, and the abscopal effect associated with a specific T-cell response in peripheral blood was present in 50% of the patients. However, the median time to progression was similar for all patients, irrespective of the antitumour immune response [27]. Although this is a controversial aspect of the study, it is unlikely that the systemic effect observed was exclusively due to the administration of intranodal rituximab, since the dose used was 100 times lower than that normally supplied intravenously when rituximab is used in monotherapy.
Conclusions
Although it is difficult to draw firm conclusions from the limited number of clinical trials conducted (which are heterogeneous and at very early stages of research), it is apparent that dendritic cell vaccines have achieved effective T-cell responses against tumour antigens, with promising clinical results, with an objective response rate exceeding 30% in patients with lymphoproliferative syndromes, a rate significantly higher than that obtained with solid tumours (0-16%). In addition, expectations have been raised by the association of these vaccines with existing immunomodular ones in the treatment of low-grade lymphomas. Future research efforts should be aimed at extending our understanding of the complex role played by the immune response in tumour development and progression, and at developing new strategies to overcome the inhibition of the immune response by tumour cells.
To read more about this article...Open access Journal of Advances in Cancer Research & Clinical Imaging
Please follow the URL to access more information about this article
To know more about our Journals...Iris Publishers
No comments:
Post a Comment