Authored by Junmei Wang*,
Abstract
Attention deficit/hyperactivity disorder (ADHD) is a neurodevelopmental disease. Amphetamine (AMP) based and methylphenidate (MPH)- based agents are the first-line medications that are used as the treatment for ADHD. In this study, we successfully constructed and validated Physiologically based Pharmacokinetic (PBPK) models for AMP of oral extended-release formation and MPH of oral immediate-release formulation and predicted the toxic and lethal doses for these two medications using our PBPK models. To create the models, we utilized drug-related parameters and system-related parameters that are obtained from database, ADMET Predictor, Simcyp built-in predictor and literature. Overall, the predicted AMP concentration in plasma was slightly underestimated but is still located within the confidence interval, while our PBPK model for MPH can predict the PK profiles very well according to the clinical data, indicating the credibility of our model. We also stated that with the application of our PBPK models, we can effectively design more rational drug regimen and estimate the toxic risk under different doses. In terms of our simulation results, we also concluded that the toxic dose of sustained-release AMP is approximately 100-400mg, and the dose over 400mg may even cause death. The dosage that is over 300mg is strongly not recommended. As for immediate-release oral MPH, the maximal recommended dose is also 100mg.
Keywords: PBPK model; ADHD; Dosing regimen; PK modeling; Stimulant; Drug abuse; Toxicity; Lethal dose
Keywords: ACAT: Advanced Compartmental Absorption and Transit; ADAM: Advanced Dissolution Absorption and Metabolism; ADHD: Attention Deficit/Hyperactivity Disorder; ADME: Absorption Distribution Metabolism and Elimination; AMP: Amphetamine; AUC: Area Under Curve; B/P: Blood-to- Plasma Partition Ratio; CLpo: In Vivo Clearance Rate; fa: Fraction available from dosage form; fu: Fraction of unbound drug in plasma; MPH: Methylphenidate; MW: Molecular Weight; NE: Norepinephrine; PBPK: Physiologically-Based Pharmacokinetic; Peff: Human Jejunum Effective Permeability; MCS: Maximum Common Substructure-based; Vss: Steady State Volume of Distribution
Introduction
Attention deficit/hyperactivity disorder (ADHD) is a neurodevelopmental disease with a prevalence of 5%-10% in children [1] and is understood to persist in adulthood in around 65% portion of cases [2,3]. Though both stimulants and nonstimulants are approved for ADHD management, stimulants including amphetamine (AMP)-based and methylphenidate (MPH)- based agents are used as the first-line clinical pharmacotherapy due to their greater efficacy [4-9]. By primarily increasing the activity of central dopamine and norepinephrine (NE), AMP and MPH exert their pharmacological effects and thereby affect executive and attentional function [12]. However, the physiological mechanism, of modulating the neurotransmitter system is also responsible for both adverse events and recreational abuse of these two drugs [10,11].
Adverse events of AMP and MPH are largely related to the stimulant-associated peripheral NE activity, whereas the euphoriant properties and abuse liabilities of these two drugs are due to their stimulation of D1 and D2 dopamine receptors, respectively [10,11]. Safety concerns with respect to peripheral NE are raised for cardiovascular outcomes, including the increase of heart rate, blood pressure, and risk of ischemic attacks, myocardial infarction, and stroke [12]. Potential abuse warnings also hasten the development and application of the prodrug, extended release or sustained release formulation of AMP and MPH, which avoids the rapid synaptic release of dopamine that produces subjective effects of a “high” similar to the effect of cocaine when these two drugs are administered intranasally or intravenously [10,11].
It’s worth mentioning that, many ADHD patients have positive therapeutic responses to oral AMP and MPH without abusing the drugs [13]. The drug abuse issue most likely happens within a certain proportion of people with a higher dopamine receptor level [10,11]. Stronger emphasis is needed for clinicians on special patient population at a high risk of abuse. On the other hand, although many adult patients with ADHD respond to dosing of AMP and MPH within the FDA-approved maximums, emerging evident has shown that some patients may tolerate or even benefit from a higher dosing that exceeds the FDA-approved ones [13]. The failure of higher doses approval by FDA is due to the lack of individualized dosing studies which prove significant benefit of such dosing regimens. Therefore, rather than sticking to fixed-dose therapy, it is of great need and importance to develop flexible and personalized dosing regimen for psychostimulants to manage ADHD. The Physiologically based Pharmacokinetic (PBPK) modeling technique has been developed and refined over the past few decades and now receives increasing attention in the process of drug discovery and development and in submissions for regulatory filing and decision making [14]. It utilizes in vitro drug data such as intrinsic clearance and bioavailability through absorption, distribution, metabolism, and elimination (ADME) as well as system data which depicts population properties to explore in vivo pharmacokinetics of drugs. PBPK models have a physiologically pragmatic compartmental structure based on the actual anatomical characteristics of the body and its organs or tissues. As a unique simulation tool, PBPK modeling can estimate the PK profile of a compound. Moreover, based on the validation by PK observations from one dosing regimen, it can predict different dosing regimens [14], providing insightful information on individualized dosing optimization.
In this study, utilizing available clinical data for patients and healthy volunteers treated with psychostimulants, we constructed and validated PBPK models for AMP of oral extended-release formulation (gelatin capsules) [15] and for MPH of oral immediaterelease formulation (tablets) [16]. By changing the value of input dose, a series of predicted PK profiles for both drugs were obtained.
Materials and Methods
Tanimoto score-based drug template selection for AMP and MPH model construction
According to our previous modeling experience, it is practicable to construct a PBPK model for a target drug with reference to a template drug which shares high structural similarity. The Simcyp Simulator (v. 19, release 1; Shefeld, UK) software for PBPK modeling has its built-in drug database where PBPK models for well-studied and commonly used drugs are available. Therefore, we first selected template drugs in Simcyp for AMP and MPH based on Tanimoto scores of these two drugs to each of the Simcyp compound within the built-in library.
Tanimoto scoring is the method of choice that is commonly used to compute the fingerprint-based similarity between different compounds. In this study, we utilized the maximum common substructure based (MCS) Tanimoto algorithm, of which the Tanimoto score is defined by the following function: Tanimoto score= C/(A+B-C), where A and B are the bits set of two compounds in their fragment bit-strings, and C is the number of common substructures shared in these two compounds [17]. The MCS Tanimoto scores between AMP or MPH and each of the template compound in Simcyp were calculated with the application of the Chem Mine similarity workbench developed by Tyler Backman, et al. In this study, a Tanimoto score over 0.5 was considered for two compounds to be largely similar.
Acquisition of PK parameters for AMP and MPH
To build the PBPK for AMP and MPH based on template drug models, AMP and MPH-specific parameters were needed. The physiochemical parameters of these two drugs were collected from the Drug bank and PubChem database. Due to the lack of literatureavailable PK parameters, we utilized the ADMET Predictor software 9.5 to predict the blood-to-plasma partition ratio (B/P) and the fraction of unbound drug in plasma (fu) of AMP and MPH, which were needed for the model construction. For system-related parameters such as the parameter of fraction available from dosage form (fa), the steady state volume of distribution (Vss) value and the in vivo clearance rate (CLpo) are based on literature report which have been shown in detail in (Table 1). Specifically, the human jejunum effective permeability (Peff) was also predicted by ADMET Predictor software.
Construction and refinement of PBPK models for AMP and MPH
In the absorption process, ADMET Predictor calculates PK parameters based on advanced compartmental absorption and transit (ACAT) model [18], with the consideration of more complicated factors like nonlinear metabolism and transport kinetics apart from multiple compartment transition rate and absorption rate. Accordingly, Simcyp has three categories of absorption model, first-order absorption model, advanced dissolution, absorption and metabolism (ADAM) model and multilayer gut wall within ADAM model. We adopted ADAM model since the it is a mechanistic physiologically based model in Simcyp which is more sophisticated than first-order absorption model. Also, the ADAM model is more like the ACAT model in ADMET Predictor software compared to other two absorption models within Simcyp. For the distribution section, we used the minimal PBPK model and its mechanism as well as model structure has been shown in (Figure 1), which displays the blood circulation among the systemic blood, hypothetical portal vein and liver compartments.
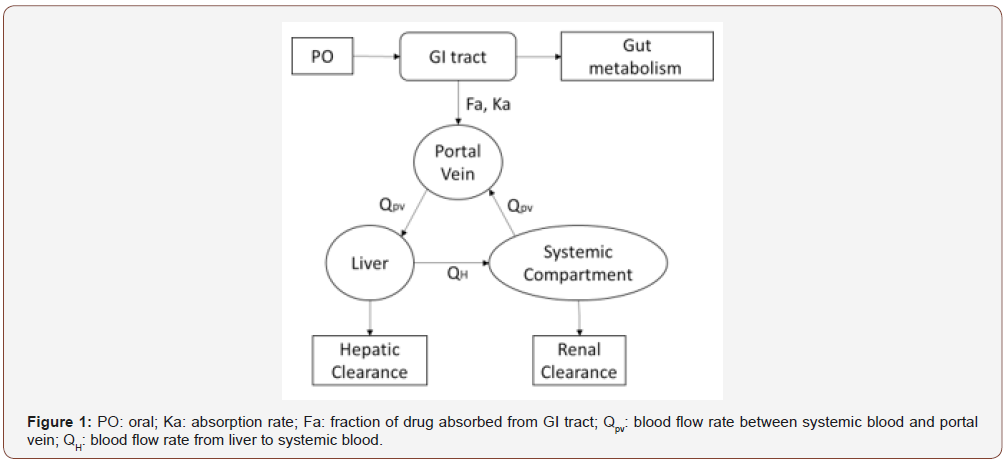
Result and Discussion
PBPK model for AMP
By comparing the drug of interest, AMP, with a series of compounds from the built-in database in Simcyp, bupropion has the highest MCS Tanimoto similarity with a score of 0.625, which is modeled with a sustained-released formulation. The clinical concentration-time profile of AMP in plasma was extracted from the literature [15]. In this report, AMP was administered orally in gelatin capsule formulation which was also one type of the sustained-release formulation, indicating it is highly likely to have similar absorption properties to the template drug, bupropion.
The collected input parameters for AMP are shown in (Table 1) (Figure 2) shows the predicted plasma concentration-time (CT) curve for the AMP model with the superposition of the clinical data [15]. From the predicted model, the Tmax is 2.88 h (Tmax from experimental data is 3.3±0.6 h), the Cmax is 0.0971mg/L (Cmax from experimental data is 0.12±0.012mg/L), and the AUC value is 1.41mg/L.h (AUC value is 1.727±0.200mg/L.h [15]). The predicted AMP concentration in plasma was slightly underestimated but is still located within the confidence interval, indicating the credibility of our PBPK model. The underestimation of our predicted concentration compared the experimental data may be explained by the different subtypes of two released formulation. Although both the AMP and bupropion are in the sustained-release formation, the former drug is reported in the gelatin capsule formation, while the latter one is of another different subtype.
Table 1: Fa: the parameter of fraction available from dosage form; CLpo: in vivo clearance rate; B/P: blood-to-plasma partition ratio; fu: fraction of unbound drug in plasma; Peff: human jejunum effective permeability.
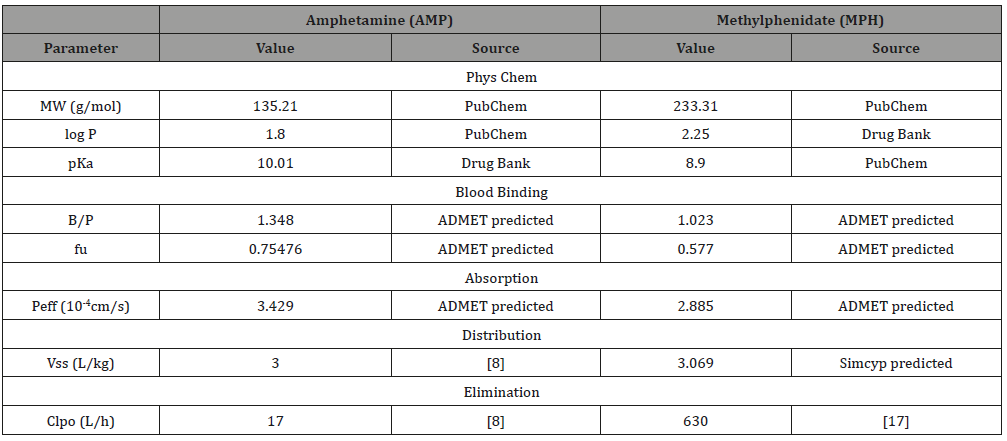
Input parameters and data sources of amphetamine and methylphenidate model
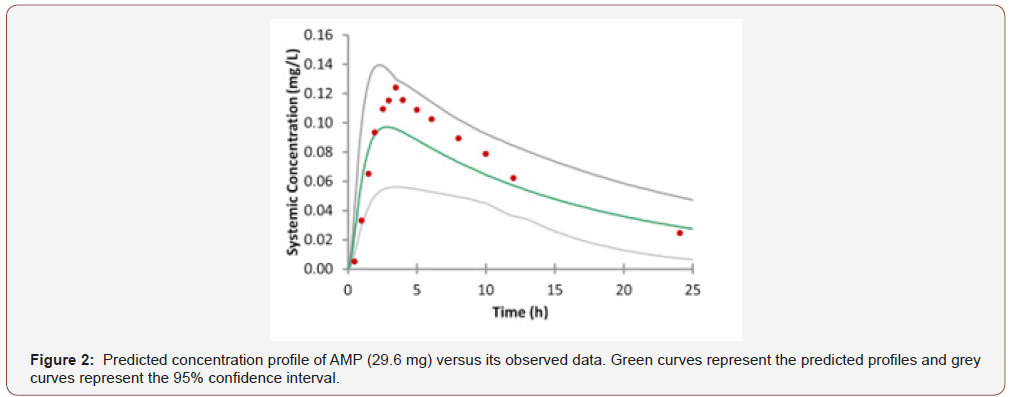
PBPK model for MPH
Although bupropion is also the most similar drug to MPH with an MCS Tanimoto score of 0.571, its sustained-release formulation is not in accordance with the oral immediate-release tablet formulation of MPH. In hence, we turned to mephenytoin, which has a second highest MCS Tanimoto score of 0.5 to MPH and a similar oral immediate release formulation. The collected input parameters for MPH are shown in (Table 1). Using mephenytoin in Simcyp as a template, a PBPK model for the target drug MPH was built and validated.
The predicted plasma concentration-time (C-T) profile for the MPH model overlaid by the clinical data is shown in (Figure 3) [16]. Overall, our PBPK model for MPH can predict the PK profiles very well in terms of the comparison between simulation results and clinical data shown in (Figure 3) For the MPH with the oral dose of 0.15mg/kg, the Tmax is 2.00 h (Tmax from experimental data is 2.2±0.4 h), the Cmax is 0.00318mg/L (Cmax from experimental data is 0.0035±0.0004mg/L). For the MPH oral dose 0.3mg/kg, the Tmax is 2.00 h (Tmax from experimental data is 2.1±0.3 h), the Cmax is 0.00635mg/L (Cmax from experimental data is 0.0078±0.0008mg/L [16]).
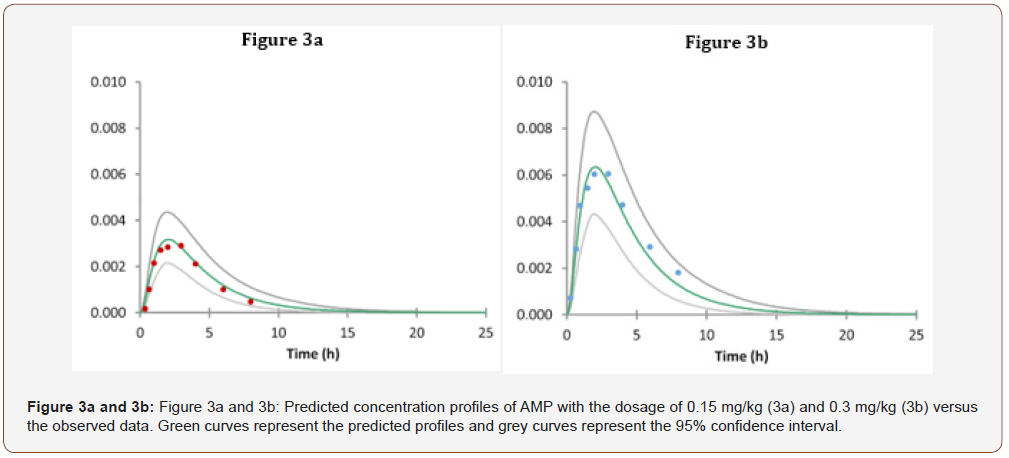
Predicted C-T profiles when adjusting doses
By utilizing the validated models and changing the input dosage of AMP and MPH, we obtained a series of predicted C-T profiles. The predicted C-T profiles of AMP with different oral dosage (40mg -500mg) are shown in Figure 4. The PK profiles of AMP, AUC and Cmax, are shown in (Table 2). As shown in (Figure 4), with the dose of AMP increased, the values of AUC and the Cmax increased accordingly. According to the report, the toxic blood concentration of AMP is 0.5mg/L, while its lethal concentration is 2mg/L. The simulation results illustrated that the upper confidence interval is close to 0.5mg/L when the dose is up to 100mg, where there might be toxic effect. At the dosage of 150mg, the average plasma drug concentration is 0.492mg/L which is very close to the toxic concentration 0.5mg/L. Dose over 300mg is extremely dangerous because its concentration at lower confidence interval has already reached 0.5mg/L. The dose at 400mg should be paid attention for its concentration at higher confidence interval, which is close to the lethal level.
Table 2: Oral dose AMP AUC and Cmax value under different dose.
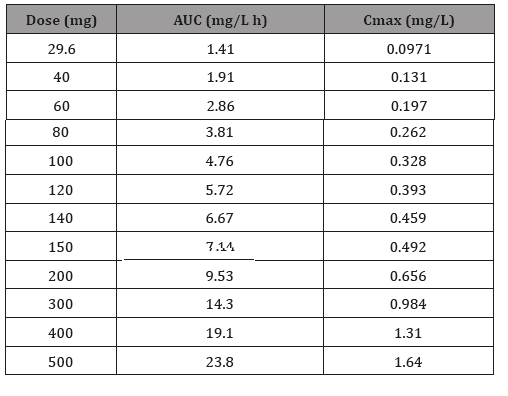
AUC: Area under curve
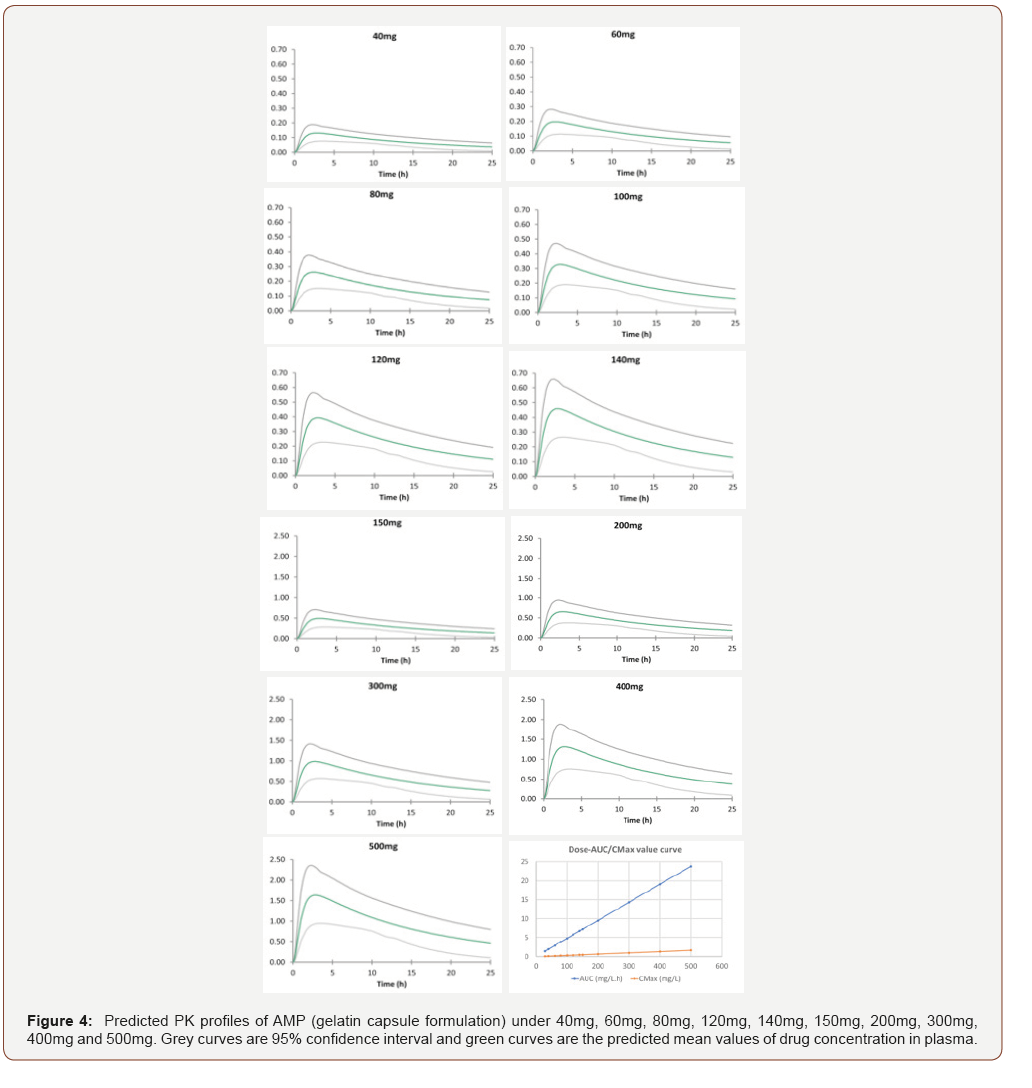
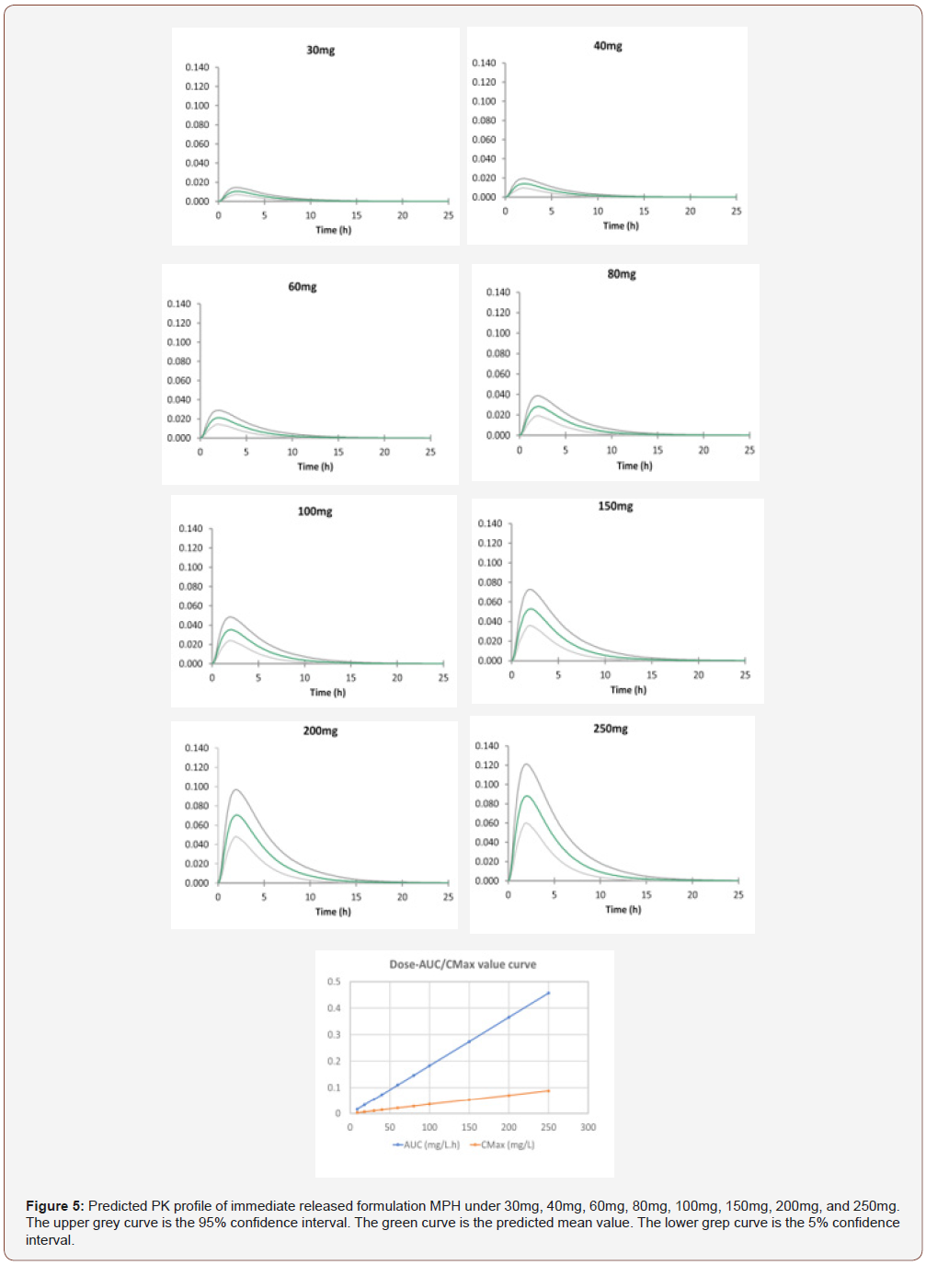
Predicted PK profiles of MPH are shown in (Figure 5) and accordingly, the PK profiles are shown in (Table 3). Other than the C-T profiles for different doses of AMP and MPH, (Figures 4 and 5) also display the relationship of AUC/Cmax versus dosage. Interestingly, the correlation coefficients between AUC/Cmax and dose for these two drugs are all close to 1, indicating the linear relationship between AUC/Cmax and dose. Due to the lack of the clinical data, the toxic drug plasma concentration for MPH is unavailable. As AMP and MPH have similar structure with the Tanimoto score of 0.5882 as well as the same stimulant effect in central nervous system, we assume that these two drugs share similar dosing properties. Because the toxic dose of AMP is 5fold to its therapeutic dose. it was suspected that the toxic dose of MPH is also about 5fold to its therapeutic dose. The normal therapeutic dose of MPH is 20-30mg/day [11], so we suggested the therapeutic dose of MPH will be no more than 100mg, where the mean value of AUC is 0.183mg/L.h. In this study, we successfully created PBPK models for AMP and MPH by utilizing drug-related parameters and system-related parameters that are obtained from database, ADMET Predictor, Simcyp built-in predictor and literature. The simulation results were validated by experiment report data, which can be further used to predict the drug concentration and facilitate the development of dosing strategy for reducing the risk of side effects of toxicity and maximizing the therapeutic effect. In addition, our reliable PBPK models can also be utilized to investigate the plasma concentration of AMP and MPH with different administration routes in different population such as pregnant women and pediatric population, promoting the design of dosing regimen and decreasing the risk of drug administration in special population.
Table 3:Oral dose MPH AUC and Cmax value under different dose.
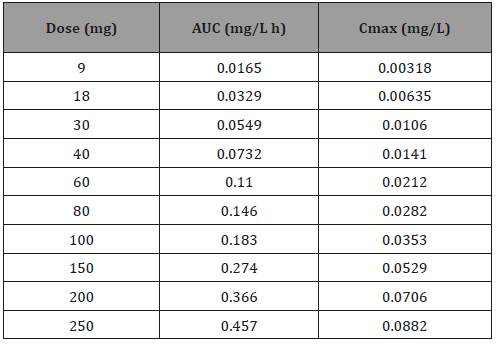
AUC: Area under curve
Conclusion
We successfully constructed PBPK models of AMP in gelatin capsule formulation and MPH in immediate released formulation to help predict their PK profiles based on reported data. Taking advantage of the PBPK model, we can help design more rational drug regimen and estimate the toxic risk under different doses. According our simulation results, the recommended maximal dose for sustained released oral AMP is 100mg. The toxic dose of AMP is approximately 100-400mg, and the dose over 400mg may even cause death. Particularly, dose over 300mg is strongly not recommended. As for immediate released oral MPH, the maximal recommended dose is also100mg.
To read more about this article...Open access Journal of Pharmacy & Pharmacology Research
Please follow the URL to access more information about this article
To know more about our Journals...Iris Publishers
No comments:
Post a Comment